Mitochondria Are Alive

The cells within our body are the remnants of an ancient alliance.
In a 1967 paper called “On the Origin of Mitosing Cells,” American evolutionary biologist Lynn Margulis proposed an idea that, upon first hearing, seems ludicrous. Her paper, in fact, was rejected by 12 different journals before it was published.
Margulis argued that one-and-a-half billion years ago, a primitive eukaryotic cell engulfed an oxygen-utilizing bacterium. But rather than digesting this bacterium — or conversely, the bacterium destroying its newfound host — the two cells gradually entered into an endosymbiotic relationship; the host provided nutrients and protection to the bacterium, and the bacterium supplied energy to the host. Margulis argued that this endosymbiosis event was a seminal “innovation engine” for biological systems, ultimately leading to the modern mitochondrion and chloroplast.
Margulis’ theory was attacked and ridiculed, igniting academic hostilities that lasted for decades. Over time, though, biologists began to accept her ideas because the membrane structure and molecular machinery within mitochondria closely resemble that of extant bacteria. Most biologists today, however, also believe that mitochondria have “devolved” into little more than membrane-bound organelles, similar to inanimate components like the endoplasmic reticulum or Golgi apparatus.
But a swelling tide of scientific evidence about mitochondrial functions and dynamics suggests otherwise — mitochondria are not just organelles, but their own life forms.
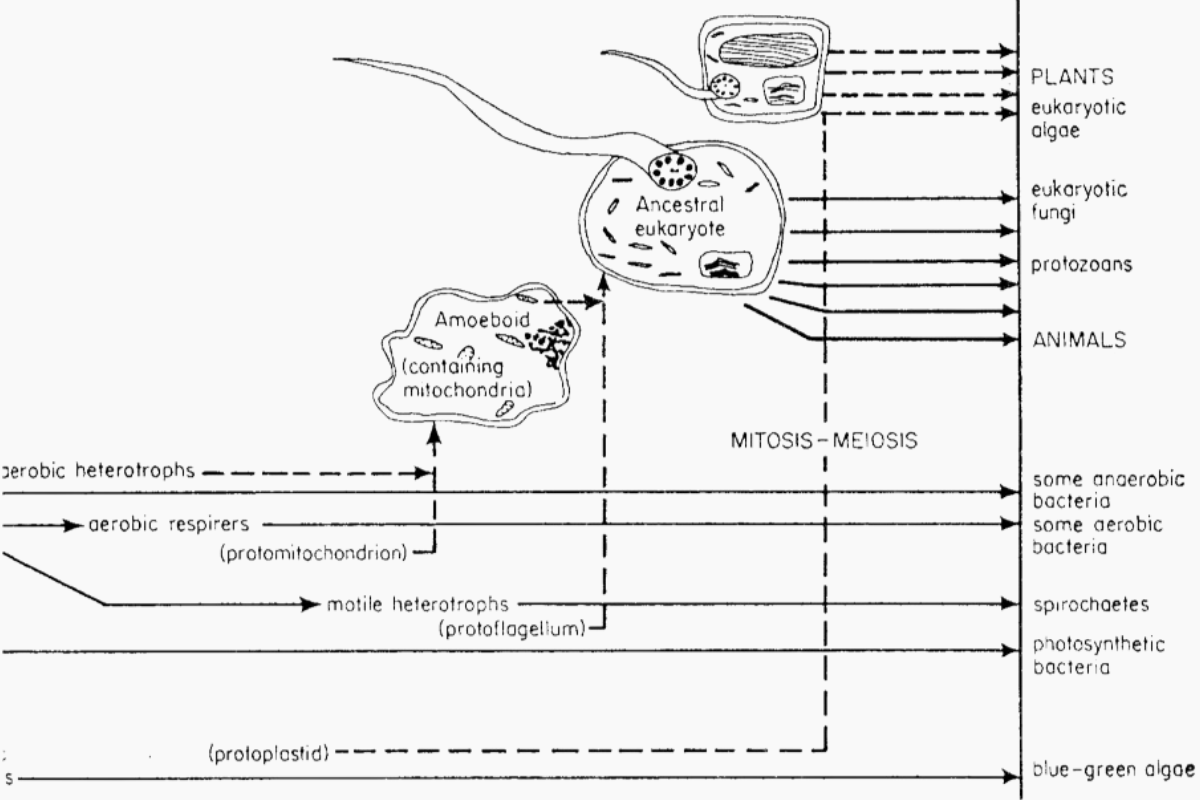
This distinction between “life” on the one hand and “mere membranous structure” on the other may seem trivial, but it’s a symptom of a deeper problem. Defining mitochondria as “nonliving” isn’t just a classification mistake, nor a question of word choice. Rather, it is a fundamental misunderstanding of the nature and role of mitochondria. It inherently undermines our understanding of biological systems and deeply influences the tools we build to study them.
If we think of mitochondria as non-living organelles, how will we ever harness their full potential?
{{signup}}
Arguments for Life
The precise definition of “life” has been debated since the inception of biology as a scientific field. Even today, researchers offer overlapping, but distinct, criteria. Molecular biologists tend to focus on characteristics like metabolism, growth and development, response to stimuli, reproduction, and the ability to process information or evolve. This definition uses “checklists” to determine whether or not an organism is alive.
Biophysicists often take a more rigorous approach, defining life by means of energetic terms. Physicists Erwin Schrödinger and Ilya Prigogine said that living organisms maintain order despite the universe's tendency towards increasing entropy, a measure of how dispersed or disordered the energy within a system is. Living systems maintain far-from-equilibrium states, constantly exchanging matter and energy with their environment to sustain highly organized structures. Cells take in low-entropy inputs, such as food or sunlight, and expel high-entropy outputs, including waste.
Regardless of which definition one chooses, mitochondria are clearly alive.
Mitochondria carry their own genomes and express their own genes within their lumens, an internal pocket of watery space, using biomolecules distinct from the cell’s nucleus. Mitochondria also replicate and divide through binary fission, much like bacteria. If one considers bacteria as living entities — and all biologists seem to — then it is impossible to explain why mitochondria are not.
From a thermodynamic perspective, mitochondria take in low-entropy inputs from their host cell, such as glucose or fatty acids, and expel high-entropy outputs, including carbon dioxide and water. Mitochondria also pump out protons through their inner membrane to maintain an out-of-equilibrium thermodynamic balance, using the resulting gradient to produce the ATP molecules that fuel cellular functions, from DNA replication to protein synthesis.
From the molecular biologist’s perspective, a mitochondrion’s role is not limited to simple energy generation, either. Mitochondria also process information and interact with their environment, much like a human cell. They monitor steroid hormones, oxidative stress, heat, ATP levels, secondary metabolites, and many more molecules floating through their environment, the cell’s cytoplasm. Mitochondria then use this information to precisely control cellular functions. For example, when a virus invades a cell, the mitochondria are critical in sensing the intrusion and signaling a host cell to undergo programmed cell death to halt its spread.
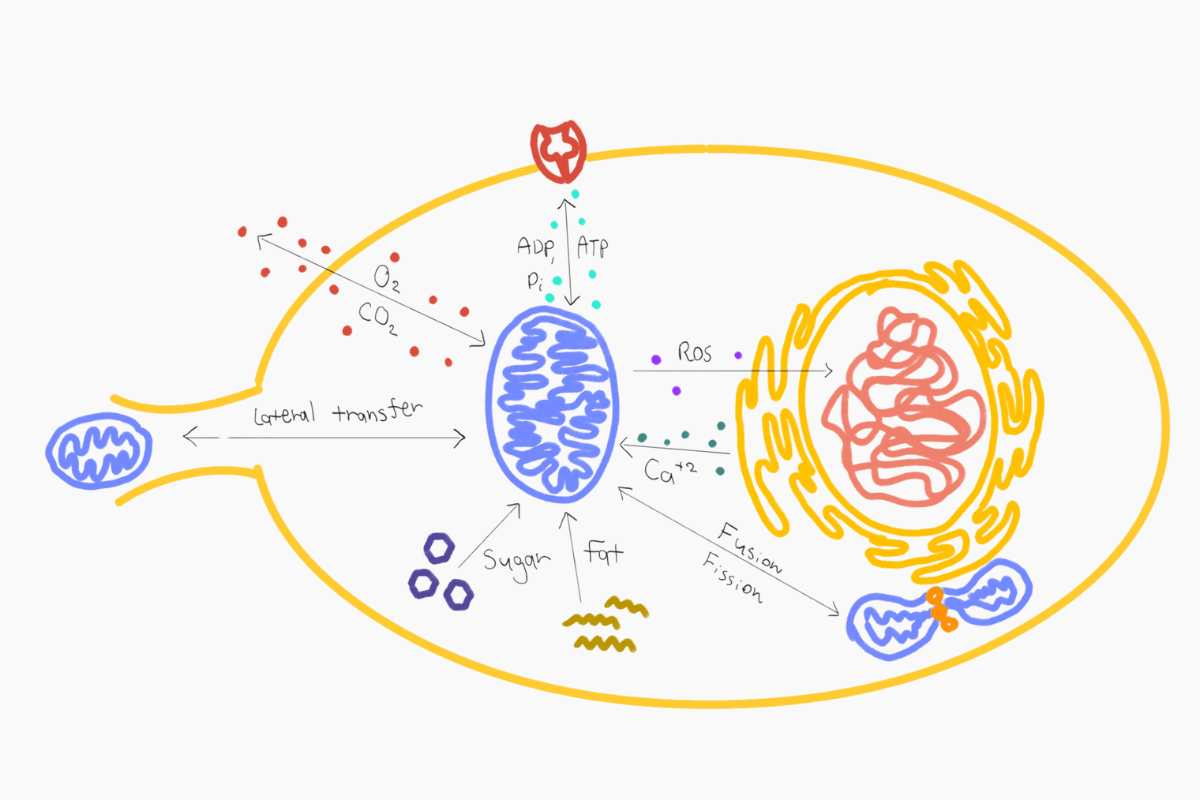
And finally, mitochondria grow and reproduce in a manner distinct from the host’s replication process. Mitochondria independently copy their circular genomes, known as mitochondrial DNA, and divide through binary fission. Notably, mitochondrial replication has several distinct properties from those observed during human cellular replication. Mitochondrial DNA mutates 100-1,000 times faster than the human genome and these mutations can significantly alter a mitochondrion’s fitness, thereby changing the fitness of its host cell. Mitochondria are thus agents of — and subject to — the forces of evolution.
Despite all this evidence, the main case made against mitochondria being alive is that they do not perform all of these functions independently, as they must be embedded within the cytoplasm of a host cell to function. However, such an argument is logically inconsistent because, by this same logic, most organisms on Earth would not be considered “living.” After all, nothing in biology lives in isolation from its environment.
Human life begins inside of another human, with a zygote requiring many months in the uterus to develop into an infant. Many other organisms — not just mitochondria — also live inside other cells. For example, the bacteria rickettsiae occupy the cytoplasm of cells of ticks, lice, fleas, and mites. Other bacteria, such as Holospora spp., also live within the nucleus of various protists. All living creatures have evolved and live embedded within an environment or biological system, with different organisms embedded in different layers.
It seems like scientists have decided what is living based on whether or not an organism exists in certain, arbitrarily chosen layers of our biosphere. But this is a logical fallacy. Every living organism grows and adapts to occupy a specific context in the universe. We refer to this as the “effective niche” of the lifeform, which could be both inside and outside of another living system. Just because an organism has evolved to live in one niche does not mean that the organism cannot survive in another. Therefore, the so-called “potential niche” of a lifeform is often much larger than its effective niche.
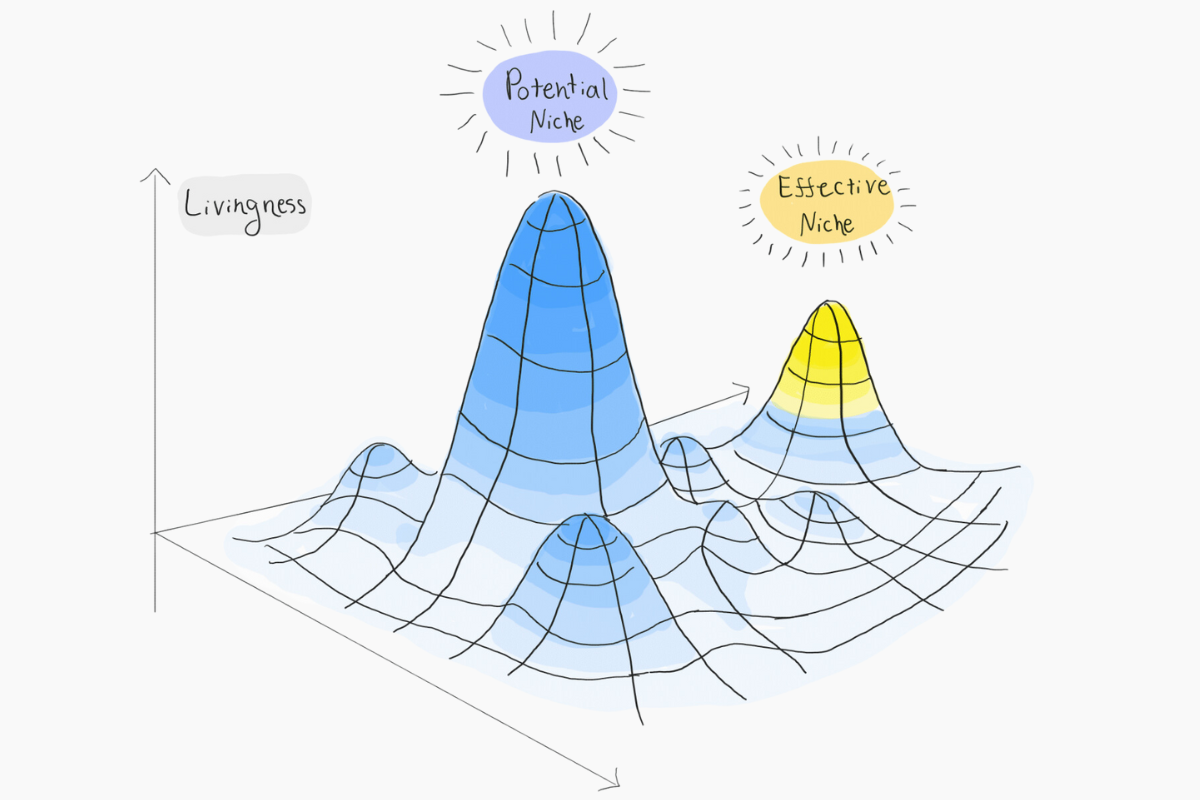
Consider, for example, that free-living bacteria have been artificially implanted into the cytoplasms of different fungi. Researchers at ETH Zurich recently implanted “bacteria into the filamentous fungus Rhizopus microsporus to follow the fate of artificially induced endosymbioses.” It is clear that the insertion of bacteria into other cells does not suddenly make those bacteria non-living.
Similarly, a mitochondrion’s effective niche is a host cell’s cytoplasm, but its potential niche is likely far greater. Mitochondria are not bound to their host cell; they can travel between different cells. Although different species carry distinct mitochondria, experiments show that mitochondria from one species can be transferred to another.
In 1997, scientists isolated mitochondria from chimpanzees and gorillas and showed that they are naturally internalized and integrated into human cells. Notably, the addition of external mitochondria even showed therapeutic benefits in heart failure and spinal cord injury. Thus, the potential niche that mitochondria can live in is greater than their effective niche.
When Margulis fought to overturn widely-held ideas in evolutionary biology, it allowed biologists to understand how complexity emerges in biological systems with the creation of eukaryotes and the rise of multicellularity. By revisiting our understanding of mitochondria, we will similarly break down long-held scientific dogmas.
A Note on Energy
In the early 20th century, Albert Einstein and Claude Shannon laid out the three pillars of the physical world: matter, information, and energy. When Francis Crick and James Watson published their model of the DNA double helix, they created a paradigm shift in our ability to understand and control the first two: matter and information. In the 70 years since then, we’ve developed powerful tools to study genes, decode how information moves through cells, and manipulate DNA using tools such as CRISPR-based gene editing. However, we have not yet reached an equal level of understanding of, or tools to manipulate, biological energy. Just as CRISPR enabled scientists to rewrite the code of life, we need similar tools to engineer mitochondria and control bioenergetics across the eukaryotic tree of life.
Despite more than a billion years of evolution, mitochondria still play critical roles within cells; they have not been displaced or rendered obsolete. This means that, as humans evolved, so too did the role of mitochondria in shaping our health and longevity. Mitochondrial dysfunction has long been linked to cardiovascular disorders, diabetes, Alzheimers, Parkinsons, amyotrophic lateral sclerosis, and other age-related diseases. In patients with these conditions, the mitochondria adopt abnormal and fragmented morphologies, failing to make enough energy for cells or sending improper communication signals. The diseased mitochondria gradually make toxic compounds that accelerate cell death.
Perhaps one of the paths to solving energy-related diseases, extending lifespan, or even engineering processes like photosynthesis lies in the complex interaction between our cells and the other lifeforms so actively inhabiting them. To find out, let’s embrace these eons-old alliances.
{{divider}}
Thanks to Kate Adamala, Zeno Fox, Michael Retchin, Niko McCarty, and Ed Boyden for helpful feedback on this essay.
Liyam Chitayat is a Hertz Fellow and PhD student at MIT working on synthetic endosymbiosis and building an initiative to integrate and accelerate the field. Liyam is also a Fellow of The Council on Strategic Risk.
Cite: Liyam Chitayat. “Mitochondria Are Alive” Asimov Press (2024). DOI: https://doi.org/10.62211/38pe-75hu
This article was published on November 8th, 2024.
Always free. No ads. Richly storied.
Table of Contents
Any part of this series can be read on its own, though the sections do build upon each other somewhat. Therefore, we recommend reading each piece in order.
Always free. No ads. Richly storied.
Always free. No ads. Richly storied.