Gregor Mendel's Vanishing Act
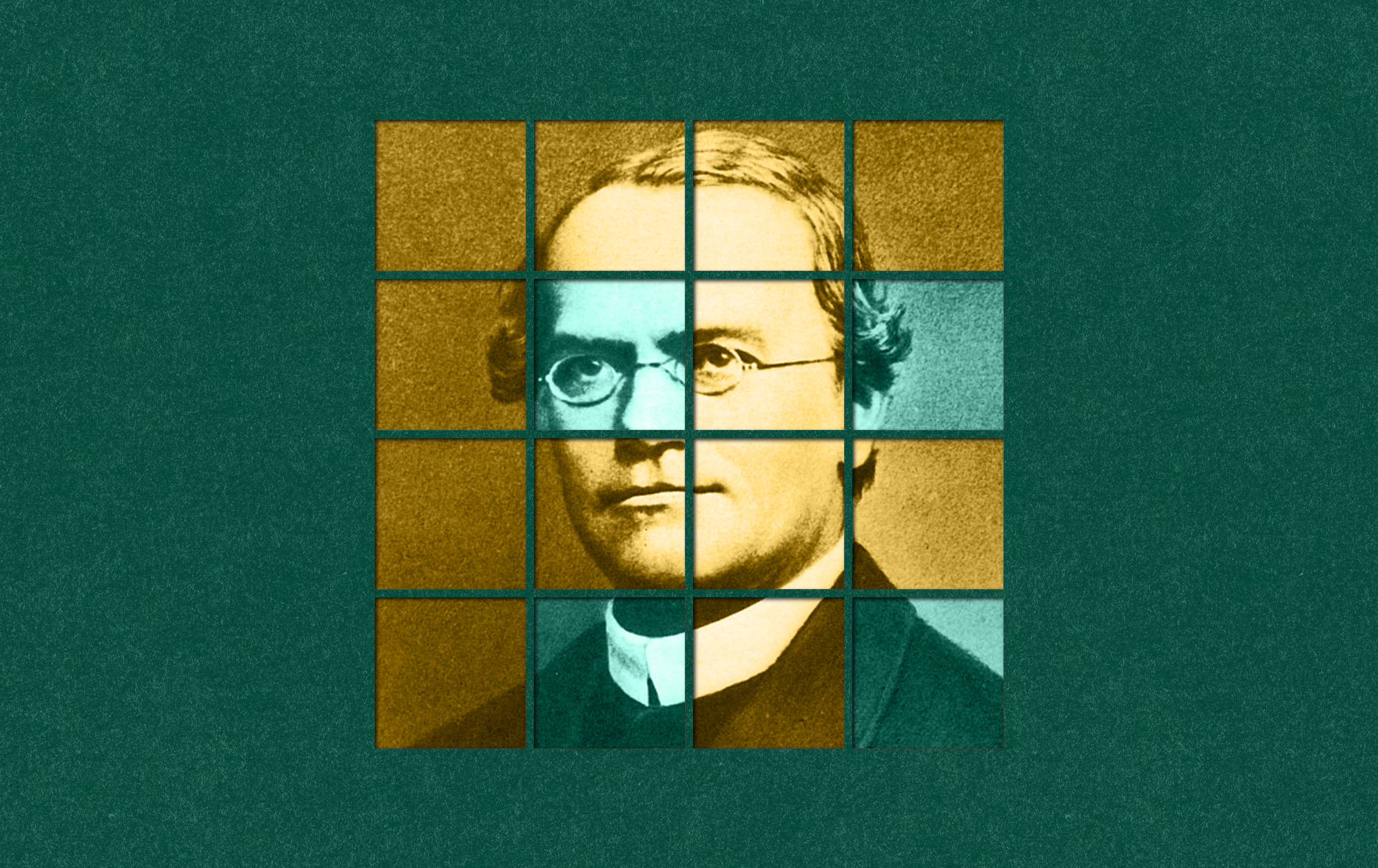
On a winter’s day in 1884, a group of Augustinian friars gathered around a fire and tossed papers into its hungry flames. Thousands of pages withered and burned, each containing hand-written text, charts, and data from a lifetime of work. By the time the smoke dissipated into an azure sky, it had all vanished.
The papers eaten by those flames had belonged to Gregor Mendel, a quiet scientist and religious man today revered as the “father” of genetics. Mendel published 14 scientific papers during his lifetime1 — mostly on meteorology and insect pests — but left behind thousands of pages detailing additional experiments, which may have amounted to a dozen or more manuscripts. We’ll never know for sure, because most of those pages were destroyed after his death. Few details survive about this event; the only source is a second-hand account recorded decades after the fact by Mendel’s first biographer, Hugo Iltis.2
This is a shame, for today much of Mendel’s work has been pigeon-holed. He has been reduced to the discoverer of a single thing — namely, the laws of inheritance. In school, students are taught about Punnett squares3 and asked to make simplistic crosses with lowercase and uppercase letters, like “aa” or “Aa.” They learn that Mendel bred peas, and little more. But the truth of this man is much deeper: Mendel was a genius of the highest order, who made important discoveries in a wide range of scientific fields.
Mendel cultivated more than 10,000 individual pea plants — and made hundreds of crosses in other plants — for his experiments. He also posited the existence of a physical material to explain inheritance, which he called “elementen,” or “elements,” many years before nucleic acid had even been isolated4 and more than 80 years before British biologists unveiled DNA’s structure and founded the field of molecular biology.

More prescient still, Mendel was the first to provide solid, numerical evidence that each parent contributes equally to the formation of offspring. Though we take this for granted today, many biologists in the mid-19th century believed in preformationism, asserting that organisms exist fully formed inside of sperm (or pollen), with the maternal eggs (or ovules) merely acting as “vessels” to nurture their growth. By doing a clever set of breeding experiments in garden peas, Mendel thoroughly debunked this idea.
And not only that. Mendel’s findings in peas, published in 1866, would later stand in stark contrast to Charles Darwin’s ideas on inheritance — though Darwin never realized it. In 1868, Darwin sought to explain inheritance using an idea called pangenesis, asserting that the cells within a plant or animal have invisible gemmules, or particles, that gather, somehow, in the reproductive organs. It is these gemmules, Darwin speculated, that transmit hereditary information to offspring. Mendel disproved this idea, too, but never published his results.
Like many biology students, I did not know about any of this until recently. In an effort to breed spicy chili peppers in my home garden, I decided to first practice on Pisum, or garden peas. I ordered pea seeds in the mail and planted them in little pots, beneath a bright rack of LED lights. Then, recalling that a friar had done much the same 160 years earlier — and in an effort to echo the work of that great experimentalist — I began reading about Gregor Mendel’s life.
The more I learned, the more I realized that my education in genetics had been sadly deficient. After devouring one biography on Mendel and then another, I plunged into his surviving letters and manuscripts before downloading and reading more than a dozen modern reviews on his life, experiments, and death. Beyond uncovering his additional contributions to the field of genetics, I learned that Mendel was plagued, throughout his life, by “nervousness” and likely from epilepsy, too. And, tragically, I learned that, while he lived, not even Mendel appreciated the importance of his own discoveries.
Many quotes in this essay derive from two biographies on Mendel’s life — notably The Monk in the Garden by Robin Marantz Henig and Gregor Mendel: His Life and Legacy by Daniel J. Fairbanks. Additional references come from Mendel’s surviving papers and letters and several academic reviews.
Despite making every effort to fully recount Mendel’s life and work, my retelling will remain incomplete due to the aforementioned bonfire; a pyre that will forever diminish the full scope of his discoveries.
{{signup}}
Fame after Failure
Gregor Mendel was born as “Johann” to German-speaking parents on 20 July 1822, in Heinzendorf bei Odrau, a small town near the Moravian-Silesian border of the Austrian Empire. His interest in plants was all but foreordained. Mendel’s father “was a peasant devoted to fruit-tree improvement by grafting,” according to a 1990 article by zoologist Paavo Voipio. By “assisting his father in the orchard, Mendel became well acquainted with the methods and problems of this field in his childhood.”
The young Mendel attended school in Troppau but often fell ill and missed classes for long stretches at a time. At the age of 18, for example, he went off to the Philosophical Institute of Olomouc to study philosophy and physics, but later took an entire year off due to illness. Because Mendel’s family was destitute, he was only able to pay for his education thanks to the beneficence of his younger sister, Theresia, who gave him her dowry.

Unfortunately, relatively few details survive of Mendel’s life. The only direct sources we have consist of 14 research papers, ten letters to a botanist in Munich (with an eleventh that is known to be missing), and a brief autobiography that Mendel wrote as a 28-year-old. There are also some surviving school and parish records. Despite the dearth of sources, we do know that Mendel’s pea experiments — and subsequent fame — were only possible because of misfortune.
At the age of 20 or 21, as his sister’s dowry funds began to run dry, Mendel joined the Augustinian Monastery of St. Thomas intent on becoming a teacher. He felt forced to do so to escape a life of poverty, later writing in his third-person autobiography “that it was impossible for him to endure such exertions any further.”
The Augustinians are members of a Catholic religious order that spend their lives improving “self-knowledge” and “searching for God.” Owing to this intellectual culture, many of the friars attended university and undertook active research programs in everything from botany to music theory. Mendel was fortunate to join the monastery based in Brünn in what was then part of the Austrian Empire (a city today known as Brno, in the present-day Czech Republic), as many of its friars were also eminent plant researchers. Upon joining the friars, Mendel chose the religious name “Gregor,” and answered to it for the rest of his life.5
In the first half of the 19th century, the Austrian Empire mandated that anyone wishing to teach in public schools attain teaching certifications. And so it was that, in his late twenties, Mendel began studying for the state board examinations to teach biology and physics at a local gymnasium (equivalent to a high school). Surviving sources suggest that Mendel loved teaching and that this may well have been his preferred path in life rather than the scientific research for which he is known today.
Mendel’s attempts to get a teaching certificate, however, were complicated. On his first examination, he passed some portions and failed others. His performance was promising enough, however, that famed physicist, Andreas von Baumgartner, recommended him for study at the University of Vienna. Baumgartner wrote to the prelate of the Augustinian friars, urging him to enroll Mendel. The prelate agreed, sending Mendel to begin his education in Vienna in 1851.
After returning from Vienna, Mendel made a second attempt at attaining his teaching certification, successfully completing the “homework” portion with excellent marks, but rescheduling the next part due to sudden illness. There is no evidence that Mendel ever completed the rescheduled examination, however, and therefore, was never certified. Mendel returned to Brünn as a supply teacher, rather than as a full instructor.
As a man who was both smart and capable, it’s unclear why Mendel abandoned the second exam. However, he was prone to what were described as nervous fits. As an Augustinian friar and friend of Mendel’s, Matthias Klácel, later wrote:
[Mendel] seems to have problems with his nerves generally since he endured several such insidious attacks already and they say that in his youth he suffered from epilepsy … One has to feel sorry for him, since his homework etc. was graded as excellent. But formalities are formalities; in this case it was not possible to continue.
Modern scientists have also suggested that Mendel may have had epilepsy. In 2022, Czech researchers exhumed Mendel’s corpse, extracted his DNA, and sequenced his genome. They found several variants in genes associated with epilepsy and neurological disorders. “He suffered throughout his life from some sort of a psychological or neurological disorder that caused him to have very severe nervous breakdowns,” botanist Daniel Fairbanks told NPR reporters.
However, if Mendel had passed his teaching exams, he likely never would have done his pea experiments or founded the field of genetics. As Vitezslav Orel wrote in his biography of Mendel:
Had he passed his teachers’ examination, he would have stayed on at the Znojmo Gymnasium, and several generations of secondary school pupils would have gained an excellent teacher. Science, on the other hand, would almost certainly have lost one of its leading discoverers.
At the university in Vienna, Mendel studied under several influential professors and, together, they shaped his ideas on inheritance, evolution, and mathematics. Mendel learned combinatorics from Andreas von Ettinghausen, and he’d later use it to rigorously examine his pea breeding experiments at a time when quantitative rigor was largely absent from botany research. Mendel also learned how to conduct hands-on physics experiments from Christian Doppler (of Doppler Effect fame), but Doppler was apparently not impressed by Mendel’s mathematical abilities. Mendel also studied plant biology under Franz Unger, a brilliant scientist who had already formulated his own ideas on evolution well before Darwin’s book, On the Origin of Species, appeared in 1859.
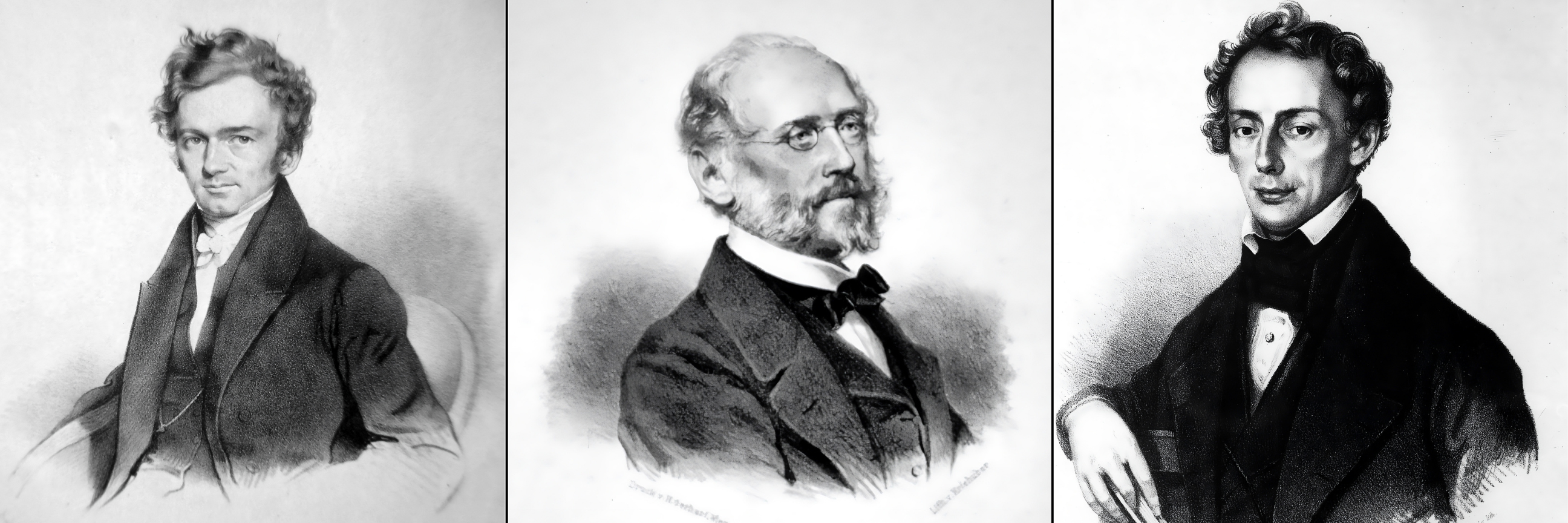
“Unger attempted to link modern plants with those in the fossil record to portray an unbroken hereditary and evolutionary tree,” writes Fairbanks in Gregor Mendel: His Life and Legacy, “branching from the ancient past to the present.”
With a few years of university studies under his belt — and now equipped with an understanding of combinatorics and the basic premises of evolution — Mendel returned to the gardens of the Brünn monastery in 1853, head aswirl with experimental ideas.
A Lucky Draw
Mendel was not the only brilliant researcher living at the Augustinian monastery in Brünn. He was surrounded by many friars of comparable intelligence who had published seminal work in their own fields. Had Mendel joined another, less intellectually-inclined monastery, his name likely wouldn’t be known today.
For example, Matthias Klácel, another friar in Brünn, performed experiments in the 1840s and 1850s that likely influenced Mendel. Klácel took alpine plants from the Moravian mountains and planted them inside the monastery walls to study whether a change in a plant’s environment could cause permanent changes in its outward appearance, or phenotype. His results were negative.6
At the monastery, Mendel also had access to a well-stocked library, including texts from legendary German scientists of the 18th and 19th centuries. Mendel read about revolutionary breeding experiments that had been performed by German botanist, Joseph Kölreuter. As Henig writes in her book, it was Kölreuter who:
… made the world’s first experimental hybrid plant by crossing two closely related members of the tobacco family, Nicotiana rustica and Nicotiana paniculata. After two years, he had converted N. rustica to N. paniculata — a feat that astounded him. ‘I did not know whether it would be a very much more remarkable thing ... if a cat were seen to emerge in the form of a lion.’
Kölreuter performed at least 65 hybridizations with 138 different species during his lifetime. He managed this feat despite sabotage from his deeply disapproving gardener, Saul. Believing Kölreuter’s experiments an affront against God, Saul would deliberately forget to heat the greenhouse and allow weeds to encroach upon the test fields.
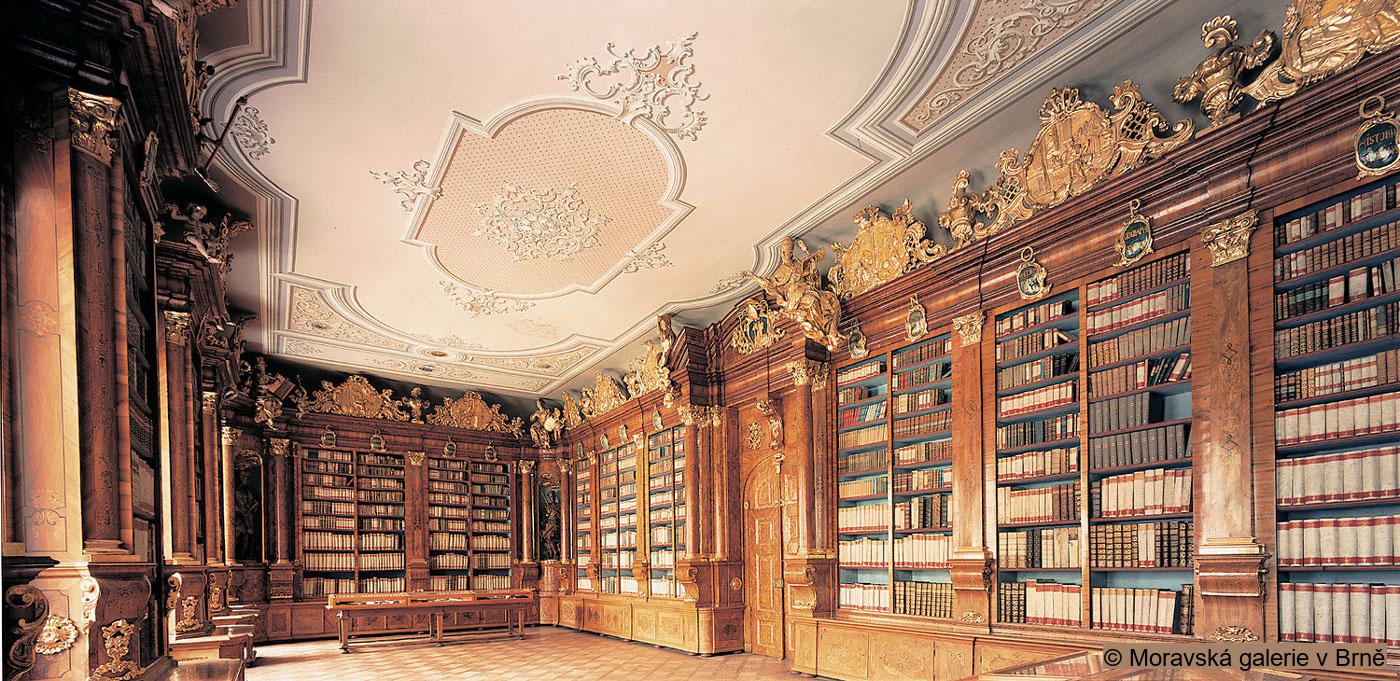
Mendel also read about another German botanist, named Carl Friedrich von Gärtner, who took Kölreuter’s experiments much further. In 1837, von Gärtner published a book revealing data from “nearly 10,000 hybridization experiments on a total of 700 species, yielding some 250 hybrid plants,” Henig writes. von Gärtner published this book at his own expense, but it seems he left out some important scientific details. As Mendel later wrote:
It is very regrettable that this worthy man did not publish a detailed description of his individual experiments, and that he did not diagnose his hybrid types sufficiently.
Between 1853 and 1856 — inspired by his German predecessors, but dismissive of their sloppy and inconsistent experimental results — Mendel began planning his now-famous pea experiments. It seems Mendel chose peas for a simple reason, as British embryologist Gavin de Beer wrote in The New York Review in 1966:
[Mendel] selected his material in such a way as to have parental pairs that differed in one contrasted character at a time. He found this material in peas, and, having crossed them artificially, he allowed their offspring to pollinate themselves for at least three generations. The results that he obtained were not only constant (whichever character was introduced through the seed-parent or the pollen-parent), but also revealed the appearance of the parental types among the offspring in different numbers which he counted and expressed as ratios.
Still, over the last century and more, biographers have felt free to pontificate on their own unfounded theories to explain Mendel’s experimental designs. Henig writes in The Monk in the Garden, for example, that:
[Mendel] kept [mice] in cages in his two-room flat, where they gave off a distinctive stench of cedar chips, fur, and rodent droppings. He was trying to breed wild-type mice with albinos to see what color coats the hybrids would have. [The bishop] seemed to find it inappropriate, and perhaps titillating, for a priest who had taken vows of chastity and celibacy to be encouraging — and watching — rodent sex.
After the bishop banned mice from the monastery, Henig claims, Mendel took to garden peas instead. A similar tale has appeared in many academic and news articles (including in Asimov Press), but it may very well be apocryphal. Fairbanks, the most serious Mendel scholar I’ve come across, says in his book that there is no evidence for it. Although Mendel published work with insect pests, and even became a renowned beekeeper late in life, banning mice would have been peculiar because the monastery’s abbot regularly bred sheep and other agricultural animals.
It is likely that Mendel undertook his pea experiments for a much simpler reason: Namely, he revered his mentor at the University of Vienna, Franz Unger, and wanted to see whether his ideas about fertilization were actually correct.
In the mid-1850s, Unger was engaged in a heated academic dispute with Eduard Fenzl, another botanist in Vienna, who believed that heredity “was purely paternal,” writes Fairbanks in Demystifying the Mythical Mendel, with “the female parent serving merely as a nurse to the pollen.” Unger disagreed with Fenzl, arguing that heredity came from both the mother and father. In other words, Unger believed that sperm (or pollen) and the egg (or ovule) contributed information equally to the offspring.
Though the answer to this debate seems obvious today, it was a heated point of contention in the mid-19th century. Mendel would definitively settle the matter and prove Unger correct — while discovering the mathematical laws of inheritance in the process.
Peas, Please
Mendel was a fastidious experimenter whose diligence bordered on obsessiveness. From 1857 until six days before his death, in 1884, Mendel recorded the air temperature, pressure, and precipitation at the monastery every single day. He repeated these measurements at 7 A.M., 2 P.M., and 9 P.M, taking detailed notes each time. Mendel sent his records to the Central Methodological Institute in Vienna for publication.

The friar applied this same level of diligence to peas. His main experiments spanned a roughly eight-year period, from 1856 until 1863. But Mendel spent the two years prior (1854-1856) ensuring that his peas were “true breeding” before initiating his crosses.7 A true-breeding plant is one that, when self-pollinated, produces identical offspring to itself. A true-breeding yellow-seeded plant, for example, will always create offspring with yellow seeds. Mendel allowed his plants to self-fertilize over several generations and then chose only those plants that consistently displayed stable, uniform traits.
To start his experiments, Mendel chose 22 true-breeding varieties to use as parents for hybridizations. Later, he whittled this number down to seven varieties with easily distinguishable traits — such as seed color, seed shape, and plant height — that are simple to observe with the naked eye.
Each cross that Mendel performed required painstaking care because pea flowers are small and delicate. To prevent the flowers from self-fertilizing, Mendel first removed the anthers with tweezers, effectively “castrating” the plant so that it produced no pollen of its own. Pea plants normally self-fertilize, since each flower houses both male and female reproductive organs. But once the anthers were removed, Mendel could transfer pollen directly from a chosen “father” plant to the emasculated “mother” plant’s stigma without any risk of accidental pollination by wind or insects. (This procedure can be viewed on YouTube.)
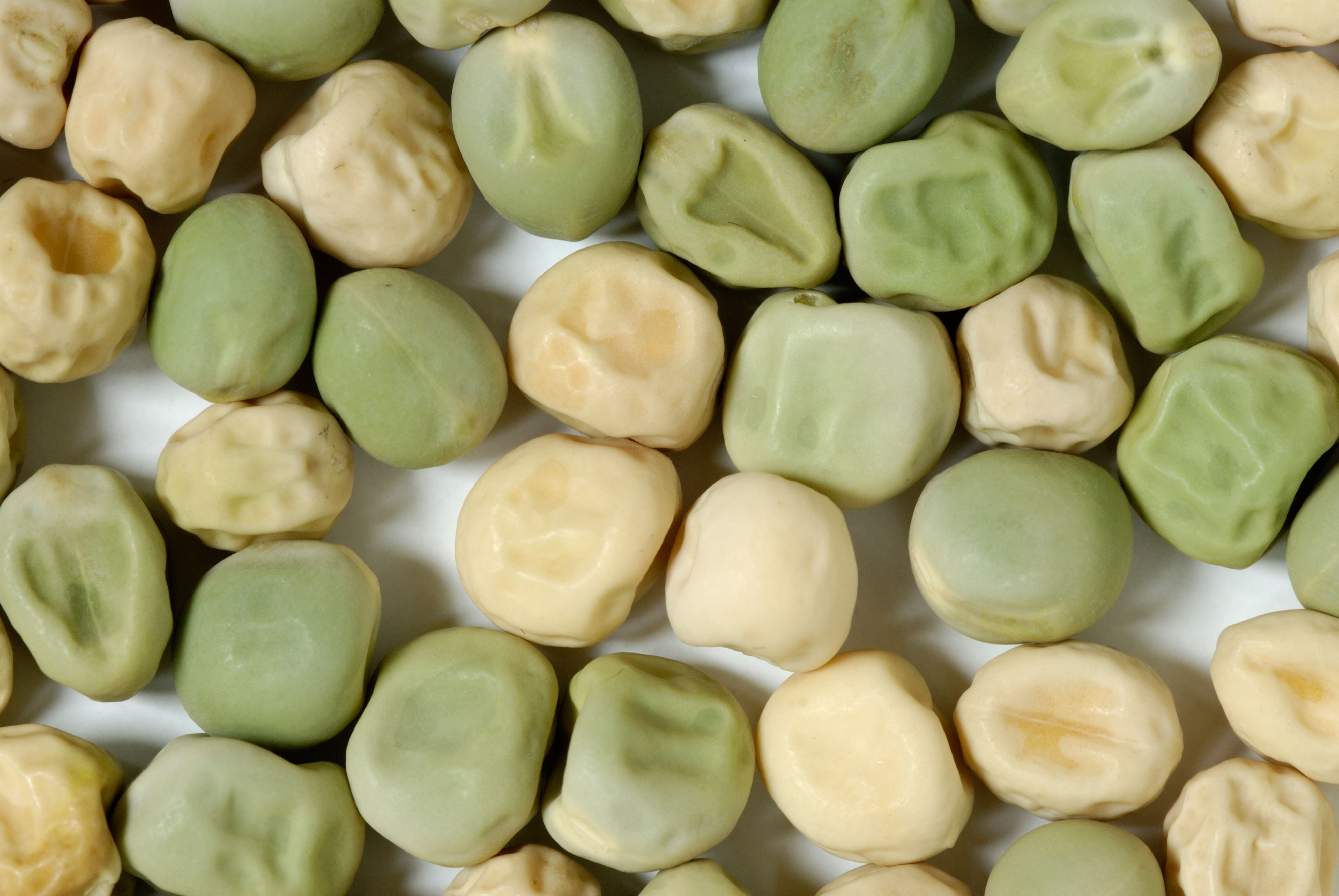
This breeding process isn’t too difficult for a handful of plants, but Mendel repeated it about 300 times over nearly a decade. He knew that he would need to cultivate many thousands of pea plants to see the neat, mathematical relationships that would later emerge from his crosses. Mendel began his experiments in 1856 with four of the seven character-pairs: seed shape, seed color, plant height, and seed coat color. He got his first results in the fall of 1856, for both seed shape and colors. For his cross on seed colors, Mendel took true-breeding plants with yellow and green seeds and then crossed the two. The plants with yellow seeds were homozygous dominant (BB alleles), whereas the green-seeded plants were homozygous recessive (bb alleles).
All of the offspring from this cross8 had yellow seeds because the yellow allele is “dominant.” They were also heterozygous; each carrying Bb alleles. Although it is obvious, at least among biologists today, that there is often a disconnect between a plant’s internal genetics and its external appearance, remember that Mendel had no way of knowing this. Plants carrying BB alleles look identical, on the outside, to plants carrying Bb alleles.
So next, Mendel did something that is nothing short of genius: He let all the offspring from his first cross self-pollinate themselves. Among the second-generation offspring that emerged, Mendel noted, 75 percent of them had yellow seeds and the other 25 percent had green seeds. In other words, the “recessive” gene for green seeds reappeared in the second generation. From these observations, Mendel began to concretize his radical ideas.
Unfortunately, the only evidence we have to understand Mendel’s experiments comes from a lecture that Mendel presented in Brünn in 1865, in addition to scattered, surviving letters that he had sent to a botanist named Carl Nägeli.
Mendel was not certain that his experiments were correct, nor did he seem to understand the importance of his work.9 But if they were correct, then they would have serious implications for evolutionary theory, which at that time was widely attacked by religious officials.
“I knew that the results I obtained were not easily compatible with our contemporary scientific knowledge,” Mendel wrote to Nägeli, “and that under the circumstances publication of one such isolated experiment was doubly dangerous; dangerous for the experimenter and for the cause he represented. Thus I made every effort to verify, with other plants, the results obtained with [garden peas.]”
Key Findings
Mendel made three additional discoveries that demonstrate his brilliance. The first of these stemmed from how Mendel designed his pea crosses to provide the evidence he needed to refute preformationism and support his mentor, Unger.
Whenever the friar bred two pea plants together, he did so in both directions. In other words, Mendel would transfer pollen from a green-seeded plant to the stigma of a yellow-seeded plant but also repeat the same cross in reverse by transferring pollen from a yellow-seeded plant to the stigma of a green-seeded plant.
My impression is that this aspect of Mendel’s experiments is rarely taught, with students hearing instead about the general principles behind his breeding experiments. But it’s key to understanding his genius; for regardless of which direction Mendel did his crosses, his offspring always appeared with phenotypes in the same ratios. Mendel thus provided the first compelling, numerical evidence that both parents equally contribute — or transmit information — to their offspring.

We take this idea for granted today only because Mendel made it obvious! In this case, it seems the friar did appreciate the importance of his results, for he wrote in his 1866 manuscript that:
With Pisum [pea] it is shown without doubt that there must be a complete union of the elements of both fertilizing cells for the formation of the new embryo. How could one otherwise explain that among the progeny of hybrids both original forms reappear in equal number and with all these peculiarities? If the influence of the germ cell on the pollen cell were only external, if it were given only the role of a nurse, then the result of every artificial fertilization could be only that the developed hybrid was exclusively like the pollen plant or was very similar to it. In no manner have experiments until now confirmed that.
A second discovery, rarely ascribed to Mendel, is evidence for the existence of a physical material of inheritance, which we would now call “genes” or “chromosomes.” Mendel asserted that there must be a physical material (he called them “elements”) that is transmitted from parents to offspring. He came to this conclusion solely by breeding plants and meticulously recording his results, and then using his mind to infer that inheritance must be transmitted through some kind of physical molecule.
Mendel’s third underrated discovery is his refutation of Charles Darwin’s faulty ideas on inheritance. In the mid-19th century, writes Henig, many biologists believed:
…that offspring take an intermediate form midway between their parents … Charles Darwin, for instance, believed in it, though the idea caused problems for his theory of natural selection. The theory states that when inheritable changes occur in an organism — and Darwin never did quite figure out how, or even how often, those changes happened — the ones that offered some selective advantage would persist, and those variants would become more and more common as the generations passed.
Darwin attempted to explain inheritance using “pangenesis.” Basically, he believed that within organisms there are little “gemmules” that travel through the bloodstream, congregate in the sex organs, and transmit information to the next generation. But this was just an idea, and “the laws governing inheritance are quite unknown,” Darwin wrote at the start of On the Origin of Species.
In search of evidence supporting pangenesis, Darwin turned to his half-cousin and fellow scientist, Francis Galton. If gemmules really existed, Galton thought, then one could presumably transfer blood between animals to change their outward appearances. When Galton tried this experiment with rabbits, though, he found that blood transfusions had no effect on the animals’ appearances (but did, occasionally, kill them.)
Undeterred, Darwin pointed to another experiment conducted by French botanist, Charles Naudin, to support his claims. In 1868, Darwin wrote:
The pollen grains of Mirabilis are extraordinarily large, and the ovarium contains only a single ovule; and these circumstances led Naudin to make the following interesting experiments: a flower was fertilised by three grains and succeeded perfectly; twelve flowers were fertilised by two grains, and seventeen flowers by a single grain, and of these one flower alone in each lot perfected its seed; and it deserves especial notice that the plants produced by these two seeds never attained their proper dimensions, and bore flowers of remarkably small size.
If information must be transmitted to offspring through a single ovule but multiple pollen grains, thought Darwin, then how could it be that one father and one mother equally contribute information to their children? Pangenesis, to him, seemed like a more likely explanation.
But Mendel took exception to Naudin’s work and set out to prove him wrong. Fairbanks describes the experiment in Demystifying the Mythical Mendel:
In 1869, while reading the chapter on pangenesis in a German translation of Darwin’s Variation of Animals and Plants Under Domestication, Mendel encountered Darwin’s supposition that fertilisation of a single germ cell requires more than one pollen grain…This passage compelled Mendel to carry out an experiment, the importance of which is evident in his description of it in an 1870 letter to Carl von Nägeli: ‘But one experiment seemed to me to be so important that I could not bring myself to postpone it to some later date. It concerns the opinion of Naudin and Darwin that a single pollen grain does not suffice for fertilization of the ovule. I used Mirabilis jalappa for an experimental plant, as Naudin had done; the result of my experiment, however, is completely different. From fertilization with single pollen grains, I obtained 18 well developed seeds, and from these an equal number of plants, of which 10 are already in bloom.'
The full results from this experiment were never published.
Renaissance Man
Today, despite his contributions to everything from beekeeping to studies on insect pests, Mendel is mostly remembered for a simple reason: He was the first botanist to seriously apply mathematics to his experiments and to carefully follow breeding patterns over multiple generations.
Mendel was also obsessively patient and diligent; traits that served him well through life. After completing his experiments with peas, for example, he followed up with hybridization experiments in more than a dozen other plant species. In letters to Nägeli, Mendel mentions hybridization experiments in hawkweeds, morning glories, toadflax, Matthiola (a type of flowering plant in the mustard family) and many more.
There were zero significant attempts to replicate Mendel's pea-breeding experiments during his lifetime and for decades after his death. Mendel was elected Abbot of the St. Augustinian Monastery in 1868. His experiments continued unabated for several years after but then began slowing down in 1873.
By 1882, at only 60 years old, Mendel was thinking seriously about his death. He prepared for it “without sentimentality, contemplating it stoically as a natural necessity,” writes Åke Gustafsson, a Swedish botanist. Prone to illness since birth, Mendel’s kidneys were inflamed, and his heart was swollen. Some scholars believe he also had chronic nicotine poisoning (“He smoked … too much, at the end of his life as much as up to 20 cigars a day,” writes Gustafsson.) “He wrote several letters to his nephews, whom he loved, in good temper, humorously, up to December 26, 1883, a few days before his death.”
On the 6th of January, 1884, at 61 years of age, Mendel died.
In his obituary, Mendel was described foremost as a beekeeper. Brünn news stories refer to the friar as an “eminent expert” and as “one of the most experienced beekeepers in Moravia.” Mendel imported bees from various parts of Europe and Africa, and even invented “innovative mating cages to ensure that queen bees mated only with the drones he chose,” writes Fairbanks, to create more productive honey bees.
Over the following months, the other friars in the Brünn monastery selected a new abbot to replace Mendel: Anselm Rambousek, another ethnic German from the Moravia-Silesia region. Nobody knows who, exactly, ordered Mendel’s papers to be burned.10 But thousands of pages — including potentially dozens of scientific papers detailing important discoveries — vanished.
Aside from the 14 papers Mendel published, we know that he had also written unpublished manuscripts thanks to quotes from his nephew, Ferdinand Schindler, who spent a great deal of time with Mendel as a young boy. In 1905 — after Mendel’s work was “rediscovered” by 20th-century biologists — a writer in Brünn went around town interviewing people who knew Mendel. Schindler told this writer (in his non-native language):
The died abbot Mendel was a man of liberal principles … He readed with the greatest interest Darwin’s works in the German translation and admired his genius, though he did not agree to all principles of this immortal natural philosopher. But it can be, that my uncle in the latter part of his life, retired from scientical evolutionary questions, because he had many clerical enemies. He said often to us nephews, that we shall find at his heritage, papers for publication, that he could not publish during his life. But we did not receive anything from the cloister, not even a thing for remembrance.
This is not the only evidence we have that Mendel’s papers were deliberately destroyed, as Fairbanks writes:
Antonín Doupovec, who attended to the aging abbot with his mother, remembered, ‘thousands of sheets of paper covered with scientific notes and data were found after his death.’ Another young friar, Pater Clemens Janetschek claimed that most of Mendel’s papers were burned after his death, only the bound books retained. It is fortunate that Nägeli and his heirs preserved Mendel’s letters. Otherwise, much of his extensive research after 1866 would have remained unknown.
Nobody knows for sure why Mendel didn’t publish more. Perhaps he had clerical enemies, as Schindler suggested. Perhaps Mendel feared retribution from the Catholic Church for publishing “heretical” ideas on evolution. Though Mendel mentions Charles Darwin in many personal letters — and, indeed, was not opposed to the British naturalist’s ideas on evolution — he never once wrote Darwin’s name in a published manuscript.
Death and Memory
Along with the oversimplification of his legacy, Mendel also suffered from the accusation of “number fudging.”
After Mendel’s rediscovery in 1900, statisticians such as Ronald Fisher noticed that his reported outcomes for the Pisum experiments seemed, statistically, too good to be true. In 1936, for example, Fisher published a paper in Annals of Science showing that Mendel’s experimental results were too “perfect,” or that they too closely agreed with statistical expectations. In other words, there wasn’t enough “randomness” or variability in Mendel’s reported results.11
Though Fisher gives the benefit of the doubt to Mendel and suggests that a deceptive research assistant may have been to blame, scholars have continued arguing these points for nearly a century now. Did Mendel really fudge the numbers?
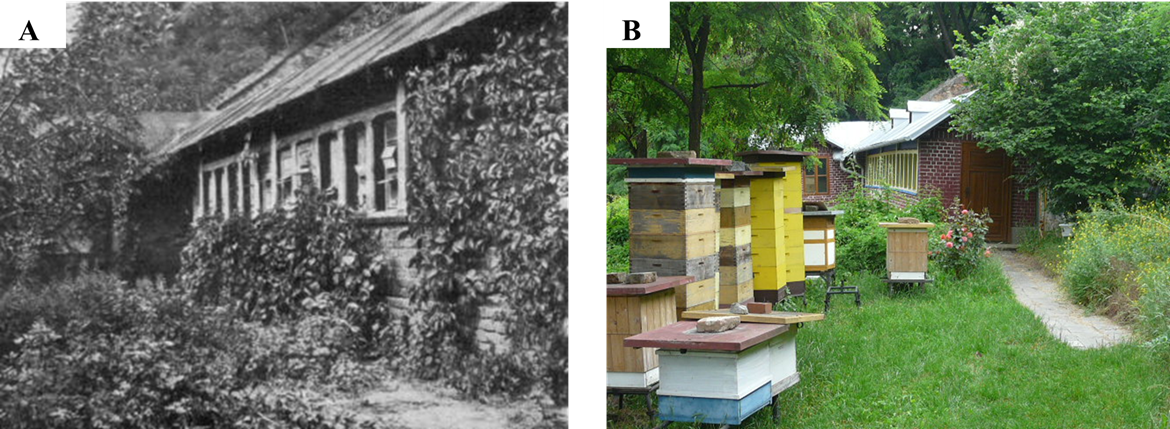
Perhaps. At the very least, it seems Mendel knew the outcomes for his pea crosses before he actually made them, as he was well-versed in the combinatorics methods he had learned from von Ettinghausen at the University of Vienna. As de Beer speculated in his piece for The New York Review:
"[Mendel] had thought out the whole scheme of ‘Mendelian’ segregation and recombination of factors in his head, as a theoretical exercise, on the hypothesis that the characters of the organism are controlled by discrete factors (we now call them genes) which exist in pairs; but the members of these pairs separate from one another (segregate) when the organism forms reproductive cells (eggs and pollen-cells), so that no reproductive cell contains more than one member of each pair of genes. At pollination or fertilization, which is random, pairs of genes are recombined. So, if one parent has genes AA and the other parent has genes aa, the reproductive cells formed by the former contain A, those formed by the latter contain a. When they are crossed, the hybrid has the genetic constitution Aa and its reproductive cells contain A or a. Now when two such hybrids are bred (or one is allowed to pollinate itself), the result is the expansion of a simple binomial: (A + a) * (A + a) = AA + 2Aa + aa."
Other evidence suggests, however, that Mendel did not deliberately “fudge” his numbers. When Mendel bred Hieracium, or hawkweed — a plant that he suspected might not follow the same inheritance patterns he had observed in garden peas — the friar made no efforts to hide the conflicting data.
Rather than go on at length, I’ll instead note that many of the biases in Mendel’s experiments can be explained, and I’d encourage curious readers to peruse the first few pages of “Mendelian Controversies,” by Daniel Fairbanks and Bryce Rytting, which lists both botanical and statistical explanations for the incongruities.
Briefly, one of the likeliest explanations for Mendel’s “excellent” results is that he simply left out a great deal of data when preparing his lectures to the Brünn Society. “Mendel made it very clear that the data reported in his paper are from a subset of experiments that he conducted,” write Fairbanks and Rytting in their 2001 article. In a letter to Nägeli, for example, Mendel referred to his 1865 paper as "the unchanged reprint of the draft of the lecture mentioned; thus the brevity of the exposition, as is essential for a public lecture.” In other words, Mendel left out some results in deference to his medium — just like so many modern biologists do today when discussing their work!
Fairbanks and Rytting continue:
"We believe that the most likely explanation of the bias in Mendel’s data is also the simplest. If Mendel selected for presentation a subset of his experiments that best represented his theories, analyses of those experiments should display a bias. His paper contains multiple references to experiments for which he did not report numerical data, particularly di- and trihybrid experiments. For example, he conducted dihybrid or trihybrid experiments for all combinations of the seven characters he studied. However, he reported data for only one dihybrid and one trihybrid experiment. In his words: ‘Several more experiments were carried out with a smaller number of experimental plants in which the remaining traits were combined by twos or threes in hybrid fashion; all gave approximately equal results’’ ... He also reported in his paper that he conducted experiments on the timing of flowering, peduncle length, and brownish-red pod color but he likewise did not report the data for these experiments."
Regardless of your stance on this debate, Mendel's was clearly a mind of the highest order. He repeated his experiments in dozens of different plants, unveiling the laws of inheritance and shattering prevailing scientific theories of his day, from preformationism to pangenesis. He predicted the existence of DNA years before it had been isolated or even revealed to be the carrier of genetic information inside cells, all while keeping bees and measuring the weather.
What is more, is that Mendel did all of this work without seeking fame or glory (even if a wish for such accolades percolated, somewhere, in the back of his mind.) Amidst the patchy paper trail of his past is a poem that Mendel wrote as a young man. Its final verse is both beautiful and prescient:
The greatest pleasure of earthly joy,
The highest destination of earthly bliss
Would be awarded me by destiny’s power
If I, risen from my grave, were to see
My art flourishing in the midst of posterity!
{{divider}}
Niko McCarty is a founding editor of Asimov Press.
Thanks to Daniel J. Fairbanks, Robin Marantz Henig, and Merrick Pierson-Smela for reading drafts of this essay.
Cite: McCarty, Niko. “Gregor Mendel’s Vanishing Act.” Asimov Press (2025). DOI: 10.62211/23pt-77gf
Lead image by Ella Watkins-Dulaney.
***
Footnotes
- Six of these papers were compilations of meteorological data, according to a 2022 article in the journal Heredity.
- The biography was published in 1924. An English translation of the original German, completed by Prof. Daniel Fairbanks, says: “Mendel's scientific legacy was not very respectfully treated. Of his notes and printed works, as Pater Clemens informed me, only the well-bound books were spared; everything else was burned.”
- Punnett squares were invented by a professor at Cambridge University named Reginald Punnett in the early 1900s, many decades after Mendel’s death. So if you despise them, don’t blame Mendel!
- Friedrich Miescher isolated DNA in 1869 after grinding up the pus coated on soldiers’ bandages. He called the substance “nuclein” and had no idea that it was the genetic material of life.
- The title of Henig’s book, The Monk in the Garden, is misleading. Mendel was not a monk; he was a friar, and there’s an important distinction between the two. As Daniel J. Fairbanks, a biologist at Utah Valley University and an expert on Mendel’s life, has written: “The mendicant orders, including Augustinians, consist of friars in that their members openly serve the community, leading much less cloistered lives than traditional monks.”
- There are cases in which a change in environment can lead to a change in phenotype, however. For the London Review of Books, Lorraine Daston writes: “Perhaps you too have planted a hydrangea in your garden, its blossom as blue as blue can be while still in its pot from the nursery, only to watch its colour muddy and turn ever pinker as the plant’s roots sink into alkaline soil. This is an example of the way the visible character of an organism can be modified by its immediate environment — in this case, soil pH. Many other instances are even more striking. The spine of the translucent water flea Daphnia shortens and ultimately disappears if it is bred for generations in polluted water, only for the long spines to reappear in the offspring of spineless Daphnia removed to clean water.”
- Mendel likely already finished his pea experiments before obtaining a copy of Charles Darwin’s On the Origin of Species. Darwin’s book was not translated into German until 1863, and Mendel did not speak or read the English language.
- The first generation of offspring are called F1 (or first filial) hybrids. The second generation is called F2. And so on.
- After Mendel completed his pea experiments, he attempted to repeat them using hawkweeds — based on the recommendation of Carl Nägeli. But the results from Mendel’s breeding experiments in hawkweed did not match up with his results from peas, a source of frustration for the friar.
- It may have also been common practice at the time, Henig told me, to burn the old abbot’s papers to make room for the new abbot’s papers.
- Fisher was not the first to make this argument. Raphael Weldon, an English biologist, used a chi-squared test to make much the same argument in 1902.
This article was published on January 12th, 2025.
Always free. No ads. Richly storied.
Always free. No ads. Richly storied.
Always free. No ads. Richly storied.