Recipe for a Cell
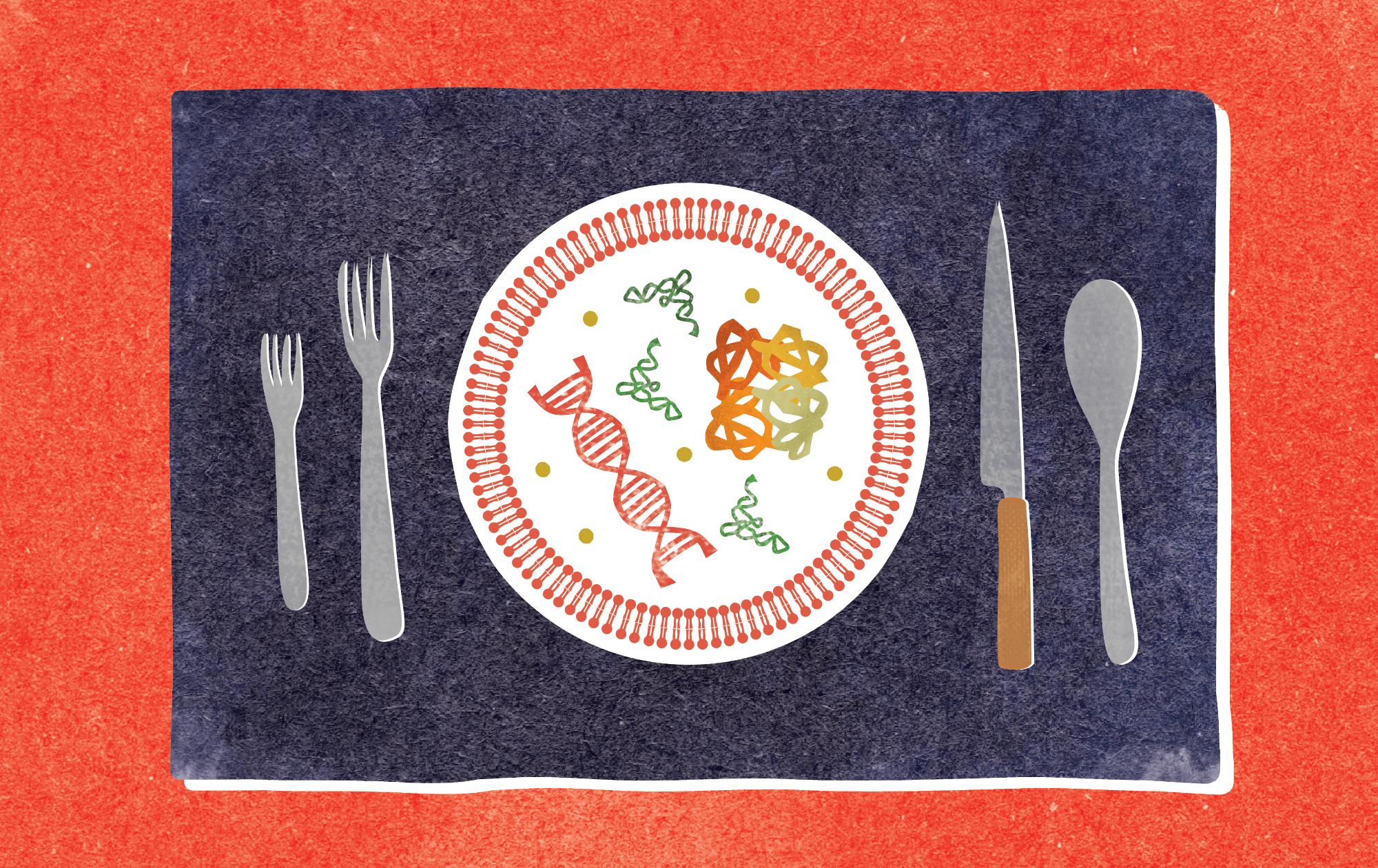
This is the second “Data Brief” in a new series quantifying biology. All the datasets used to create this article are freely available on GitHub.
{{divider}}
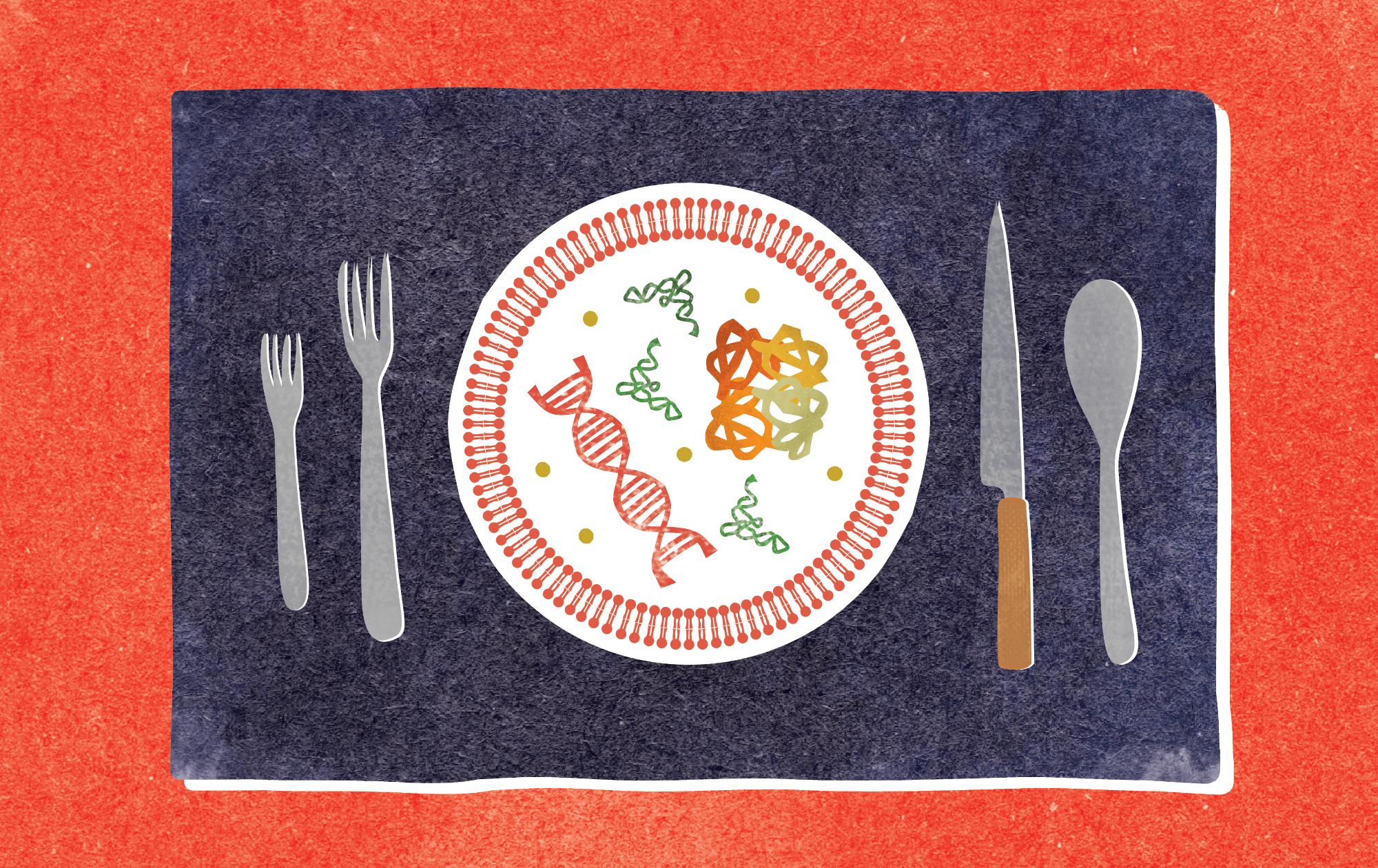
Biologists have long pondered whether life can be reduced to discrete chemical components or, conversely, whether those same components could be used to assemble an organism from scratch. Perhaps in the future, human hands might tailor a cell by stitching together individual molecules with the world’s finest sewing machine. But for now, this remains a distant dream.
Fortunately, cells can accomplish on their own what humans can’t. Living organisms routinely assemble new lifeforms from scratch (a.k.a. their offspring) by converting raw inputs like sugars, nitrogen, and energy into highly ordered structures, beating back the unceasing tides of entropy. Or, as the Austrian physicist Erwin Schrödinger wrote in his 1944 classic, What Is Life?, cells refuse to succumb to chaos “by eating, drinking, breathing and (in the case of plants) assimilating.”
While we humans can’t yet perfectly replicate cellular function, we can engineer, co-opt, and coerce cells to do so on our behalf. The entire premise of synthetic biology is that cells are “programmable” machines capable of nano-scale fabrication, and that, furthermore, one can engineer those cells to create medicines, foods, and materials.
Any effort to engineer a cell, though, should begin with a catalog of its parts. What are a cell’s necessary components? How many atoms — and how much energy — does it take to build a new one?
It’s easiest to answer these questions for the bacterium, Escherichia coli, because that microbe has been studied more than any other. It is the subject of more than 100,000 published research papers. Scientists have marveled at “flesh-eating” E. coli and engineered strains that act as living “camera film.”
But even using a single, well-characterized species like E. coli as a guide, there’s no one-size-fits-all cellular template. The size and shape of each bacterium changes a great deal depending on its environment. When E. coli cells have abundant access to nutrients, they tend to be larger. If nutrients are scant and conditions are poor, they become smaller and divide more slowly. In general, however, the mass of an E. coli is about 1 picogram, or one one-trillionth of a gram. That’s astonishingly small, roughly equal to the weight of the DNA inside a single hummingbird cell.
Which molecules make up this mass?
{{signup}}
About 70 percent is just water. The other 30 percent — the so-called “dry mass” — is composed of everything else: DNA, RNA, proteins, lipids, and ions. Surprisingly, DNA accounts for just 3 percent of this dry mass, yet encodes all the information needed for the cell to grow, divide, adapt, and evolve. Most of a cell’s dry mass (55 percent) is proteins, which dominate because they are the “executors” of cellular functions; they catalyze reactions, form structural scaffolds, and regulate gene expression. About 20 percent of the dry mass is RNA, 10 percent is lipids, and the rest is ions and signaling molecules.1
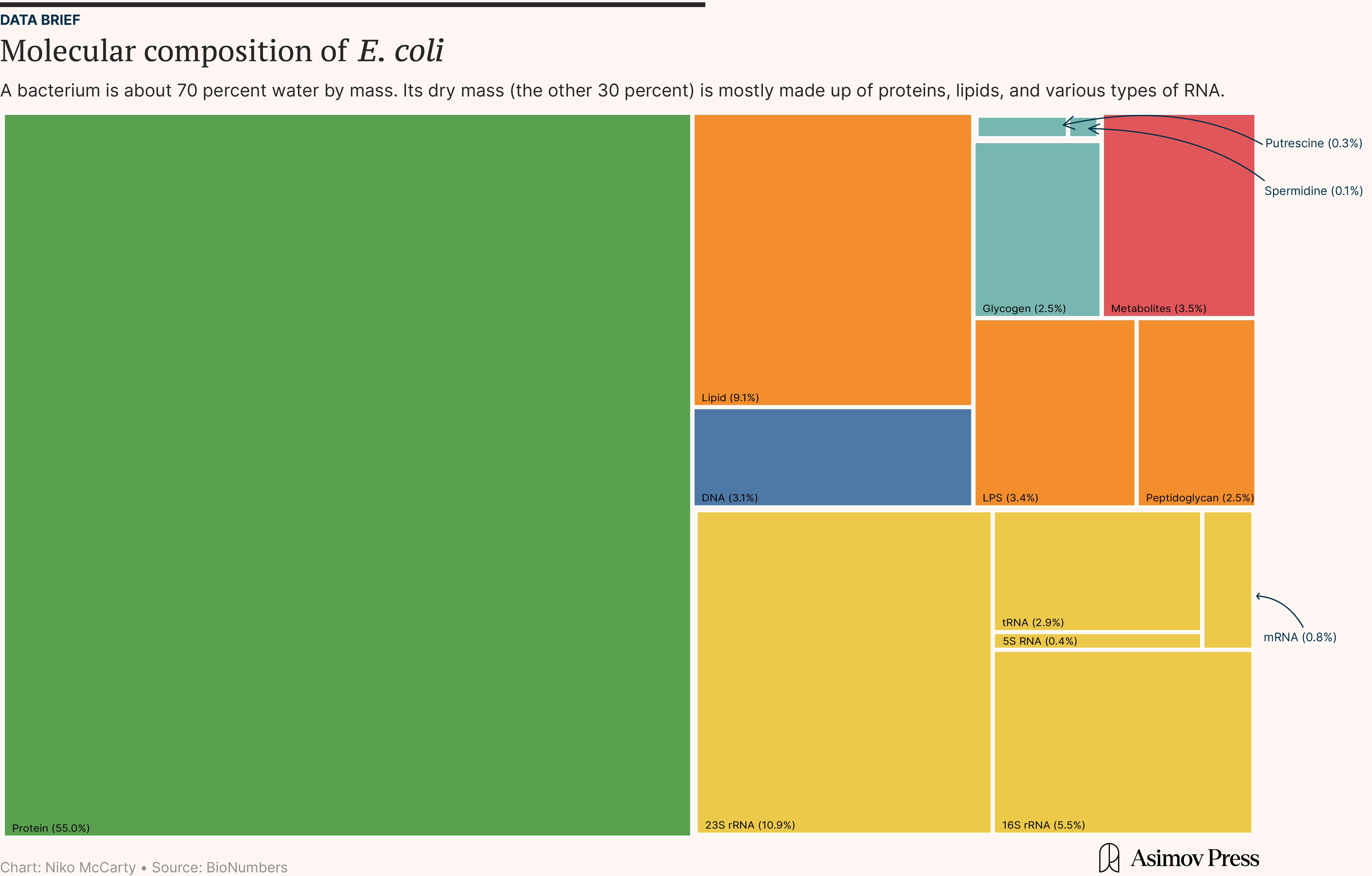
It’s worthwhile to pause for a moment and consider why proteins form the bulk of a cell’s dry mass. And the simple fact is that there are lots of them, and each protein is relatively heavy. A single E. coli cell packs upwards of two million proteins into its tiny body, and each protein contains dozens to hundreds of amino acids. A typical 300-amino-acid protein weighs around 33 kilodaltons (kDa), equivalent to the mass of 50 base pairs of DNA.2
A small number of protein types also account for the vast majority of all proteins in a cell. In 1978, researchers believed that EF-Tu — a protein that works with ribosomes to synthesize proteins — was most abundant, with a few hundred thousand copies per cell. Then, in 1979, a paper in Cell made the claim (still held today) that the most abundant protein is Lpp, with over 700,000 copies per E. coli cell. Lpp holds the outer membrane to the peptidoglycan layer, preventing cells from collapsing. Without this protein, a cell would be crushed by its turbulent surroundings.3
All of this points to a final question: Namely, how much energy would it take to engineer these components from scratch?
One might assume that it would take a lot. After all, there are millions of proteins per cell, each crafted by stitching together individual amino acids into long chains. It requires a great deal of energy to make each amino acid and then combine them. Similar considerations apply for DNA, RNA, and all the other molecules, too.
But the actual amount of energy required is surprisingly small. Scientists recently used computational models to estimate the energy cost of assembling every part of a single cell. They first used experimental data to quantify E. coli’s molecular composition, and then applied a group-contribution algorithm to calculate the standard Gibbs free energy required to form each type of biomolecule. By adding together all these calculations, the researchers arrived at the minimum energy required to build an E. coli: 9.54 x 10-11 joules.
Interestingly, constructing the lipid bilayer — the membrane that partitions a cell from its environment — turns out to be the second most energetically expensive part of the cell to create, even though lipids account for less than 10 percent of dry mass. This is because lipids are made from long carbon strings fused together via high-energy ester linkages, which requires more Gibbs free energy per gram to synthesize than any other type of biomolecule (even proteins).
While we now have a nearly complete catalog of E. coli’s molecular parts — and estimates of the energy required to assemble them — building a functioning cell from scratch still remains one of biology’s most elusive goals. The challenge is not merely mechanical, either. Even if one gathers all the right molecules in the right quantities, arranging those molecules with sufficient precision to “create life” remains far beyond current capabilities.
This is, in part, because cells are dynamic. They move and change quickly. Sugar molecules fly through a cell at 250 miles per hour, and each protein is bombarded by 1013 water molecules every second. This dynamism is often overlooked in the methods used to study living cells. A list of ingredients is inherently static. The next step, then, is to capture how each piece moves and interacts. Only then might we turn our catalogs of parts into recipes for a cell.
{{divider}}
Niko McCarty is a founding editor of Asimov Press.
Cite: McCarty N. “Recipe for a Cell.” Asimov Press (2025). DOI: 10.62211/34pk-99hr
Lead image by Ella Watkins-Dulaney.
Correction: An earlier version of this article included an incorrect calculation on the energy required to build an E. coli cell.
Footnotes
- Once we know the macromolecules that make up an E. coli cell, we can also estimate the raw elements — or even chemical formula — used to build such a cell. A typical E. coli cell contains about 1010 carbon atoms and has a chemical formula of C4.4H7.2O2.1N0.8P0.086S0.039. This means that for every 4.4 carbon atoms, the cell has 7.2 hydrogens, 2.1 oxygens, 0.8 nitrogens, and a tiny fraction of sulfur and phosphorus. This formula is derived by averaging the approximate composition of proteins, nucleic acids, lipids, carbohydrates, and other cell components.
- One kilodalton is equal in mass to 83 carbon-12 atoms.
- A 2023 paper in Science Advances used atomic force microscopy to visualize Lpp in individual cells and concluded that each cell contains hundreds of thousands to about one million copies.
Always free. No ads. Richly storied.
Always free. No ads. Richly storied.
Always free. No ads. Richly storied.