The Origins of Adjuvants

James Phipps, the son of a poor, landless gardener, was only eight years old when his father signed him up for a risky experimental procedure to be performed by his employer, the British physician Edward Jenner. It’s unclear whether his father fully understood what he was consenting to or whether he had been persuaded by a payment. Either way, young James set out for the doctor’s home to receive the world’s first vaccine.
A lithograph from the period depicts what unfolded next. James squirms for freedom as his father restrains him against a chair.1 Although not captured in the lithograph, we know that, before administering the vaccine, Jenner scratched the boy’s arm with an implement until it bled and then plunged a lancet smeared with cowpox taken from a milkmaid into the wound.
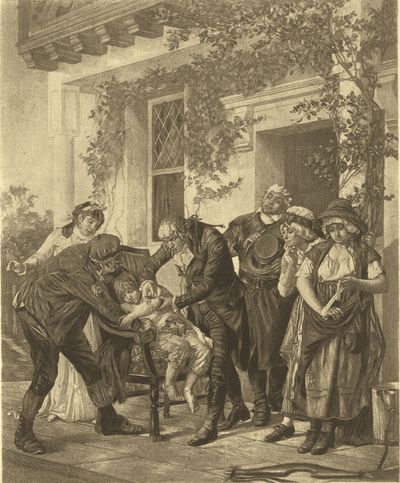
That first vaccination occurred on 14 May, 1796, at a time when smallpox infected about 1.3 million people in Europe every year, killing 30 percent of them and blinding one-third of the survivors. People who had been infected with the virus were easily recognizable, for they carried scars where inflamed pustules had once blistered. In 1746, the London Smallpox Hospital compared the scourge to “a thorny hedge through which all must pass, and some die, to reach a field of safety.”
Smallpox sufferers spread the virus to between three and six other people on average. No one, it seemed, was immune, and after the inoculation of young Phipps, Jenner was quickly celebrated as the inventor of vaccination. Some sources even heralded him as the “father” of immunology. And although Jenner’s invention would, indeed, lead to the eradication of smallpox, this truncated story neglects the contributions of many other scientists whose work has made modern vaccines safer and more effective.
The next generation of vaccinologists worked to design effective immunizations against a multitude of pathogens. However, many early vaccines did not trigger immunity; single subunit vaccines containing one piece of a pathogen were particularly likely to fail.
A breakthrough came more than a century after Jenner’s work, when 20th-century scientists discovered chemicals called “adjuvants,” which stimulate the immune system and enhance their reactions to vaccines. These have proved essential for bolstering immunizations, particularly subunit ones, and though many people have never heard of them, they benefit almost everyone who takes vaccines. Indeed, half of all vaccines used today contain an adjuvant called “alum.”
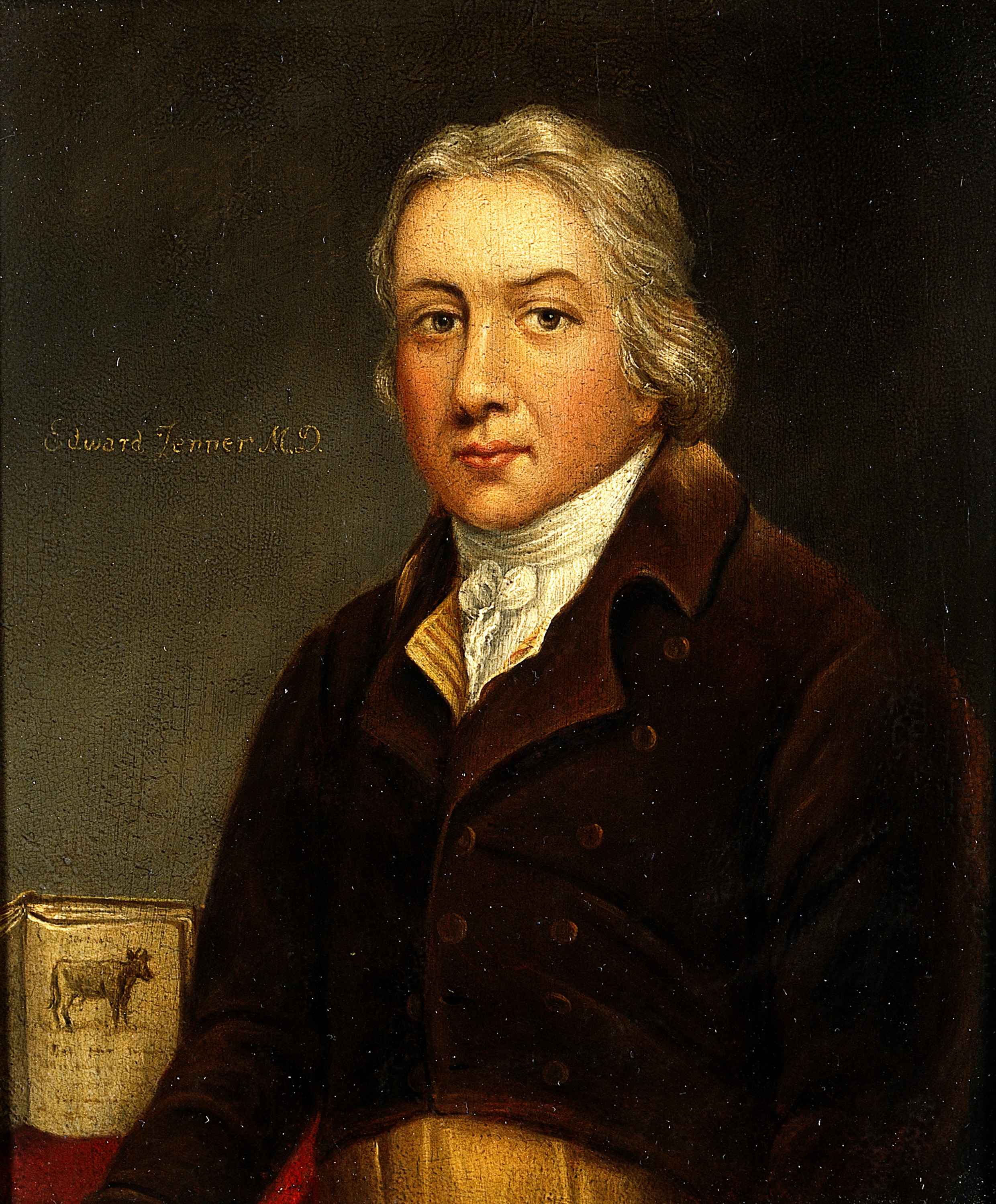
Adjuvant research experienced a huge boom in the early 20th century. Today, six adjuvants have been approved by the Food and Drug Administration (FDA), each triggering a different kind of immune response. Still, this handful of adjuvants does not provide nearly enough variety to stimulate distinct immune reactions, and a trove of molecules — from plant extracts to synthesized nanoparticles — with the capacity to trigger different defenses awaits investigation.
{{signup}}
Long Shot
In the 18th century, before vaccines, infections were — by far — the leading cause of death. In England, smallpox alone “caused about half of the deaths of children under age ten,” writes Jason Crawford, founder of the Roots of Progress Institute. Smallpox was so feared that “no man dared to count his children as his own until they had the disease,” the French explorer Charles Marie de La Condamine once asserted. Crude as it was by today’s standards, Jenner’s vaccine played an enormous role in curbing the disease’s spread.
After physicians tested out Jenner’s vaccine on 160 other people, demonstrating its safety and effectiveness, it quickly became popular among the upper class. Jenner also started vaccinating the poor for free in his “Temple of Vaccinia,” a small thatched hut in his garden. After much campaigning, people scrambled for it: Public posters heralded Jenner’s invention as “the only security against this dreadful malady,” and one newsletter reported that “the advantages which the Jennerian, or Vaccine Inoculation, will secure to mankind, are incalculable.”
Still, vaccine skeptics existed even then, with some erroneously suggesting the cowpox inoculation would cause recipients to grow cow parts. By the late 1800s, English law had overruled them, making smallpox vaccines compulsory, and deaths from smallpox dropped dramatically. Between 1889 and 1898, the disease caused only 10 deaths in England. Other countries also adopted the vaccine during the late 1800s and, by 1980, smallpox was deemed eradicated.

Prior to vaccines, people hoping to avoid severe smallpox symptoms had few options and typically resorted to a primitive inoculation method called variolation. This technique, possibly used as early as the 10th century in China, was more dangerous than vaccination because it employed deadly smallpox as opposed to milder cowpox — a relative of smallpox that usually infected cattle, and occasionally caused mild symptoms in people, such as blisters on the fingers. Variolation involved scraping infectious matter off a dried scab from a smallpox patient, smearing it onto a needle, and then pricking the skin of an uninfected person to pass on the virus.
Variolation reduced the risk of death from smallpox for two reasons. First, the smallpox virus caused less severe disease when contracted artificially from a dried scab rather than naturally from inhaled air droplets, potentially because less virus survived inside the desiccated tissue. Second, people could only contract it once, which we now know was due to long-lasting immune memory. Those who survived the milder form of disease induced by variolation gained life-long immunity. As a result, the practice grew in popularity and spread to Europe by 1721.
Even as it offered some protection from the disease, the technique sometimes produced disastrous results, killing about 3 percent of its recipients. While this might not sound like much (and, indeed, deaths from variolation were ten times rarer than from natural smallpox infection), variolation was risky compared to vaccination, which caused virtually zero deaths.2
In 1768, during variolation’s prime, an English surgeon named John Fewster noticed that some of his patients had pre-existing immunity against smallpox. Fewster spoke with a farmer, who said, “I have had the Cow Pox lately to a violent degree, if that’s any odds.” When Fewster followed up with other dairy workers, he discovered that all of them had suffered cowpox — they contracted the virus on their hands by milking cows with viral lesions on their udders. Fewster realized that cowpox infection somehow protected people from the “speckled monster.”
Many retellings of the smallpox vaccine’s origins neglect to mention Fewster’s contribution. Instead, they mislead readers into thinking Jenner was the first to notice the connection between cowpox and smallpox by observing that “beautiful milkmaids” who suffered cowpox never caught smallpox, which could have permanently scarred and disfigured their otherwise captivating faces. As Crawford has written:
[Fewster] reported the finding to a local medical society, and eventually it became known to a nearby surgeon’s apprentice named Edward Jenner. (The origin story that is usually told, where Jenner learns of cowpox’s protective properties from local dairy worker lore or his own observations of the beauty of the milkmaids, turns out to be false — a fabrication by Jenner’s first biographer, [John Baron] possibly an attempt to bolster his reputation by erasing any prior art.)
Jenner did, however, seize the opportunity to test whether cowpox inoculation actually shielded against smallpox during an outbreak in 1796. He sampled cowpox from Sarah Nelmes, a dairymaid. Using a lancet knife, Jenner extracted pus from a cowpox blister on Nelmes’ arm and, after scratching James Phipps’ arm with a separate knife, swiped the infected pus from the lancet into the boy’s wound. Phipps fell only mildly ill, experiencing some soreness and a fever before swiftly recovering. He also didn’t pass the virus on to the other children with whom he shared a bed.
Jenner’s experiment suggested that vaccination offered fewer risks than variolation. But the clincher came when, after Phipps’ recovery, he returned to Jenner’s home to receive smallpox inoculations on at least 20 different occasions. The young boy never contracted the disease. Jenner never learned why cowpox protects against smallpox infections, but studies in the 20th century confirmed that antibodies against cowpox can detect and neutralize smallpox due to similarities between the viruses, thus clearing smallpox from the body before the disease can gain a foothold.3
Successive live-vaccines built on Jenner’s model, such as those for measles, mumps, rubella, influenza, tuberculosis, and the oral polio vaccine. But whereas Jenner lacked the experimental tools to tamper with the cowpox virus’ properties before inoculating patients, researchers can now alter the genomes of live pathogens, either by artificial selection or gene editing, to render the microbes harmless. Other vaccines, including those for hepatitis A, polio, and rabies, use whole microbes killed using chemical fixatives or heat. Live-attenuated or inactivated vaccines generally prove effective because the microbes carry enough foreign material to alert the immune system to their presence.
A whole microbe, dead or alive, doesn’t always make the best candidate for vaccination, however. Some bacterial infections, like diphtheria or tetanus, cause disease using protein toxins, so the immune response must narrowly focus on neutralizing those protein targets. This led to the invention of subunit vaccines in 1923, which include only a piece — or “subunit” — of a pathogen. However, without the flurry of immune-stimulating molecules present on whole bacteria, such vaccines usually triggered only weak immune reactions.
To gain potency and fulfill their potential, vaccines awaited another ingredient.
A Horse of a Different Calor
Gaston Ramon, a French veterinarian living near Paris in the early 20th century, provided it. Ramon began his career working on an immunization method called serotherapy, but his research would eventually lead him to discover adjuvants, forever improving vaccines.
For serotherapy, Ramon injected horses with tetanus or diphtheria toxins. The horse immune system made antisera (i.e. antibodies) against the molecules in response, which Ramon later collected to treat infections in humans.
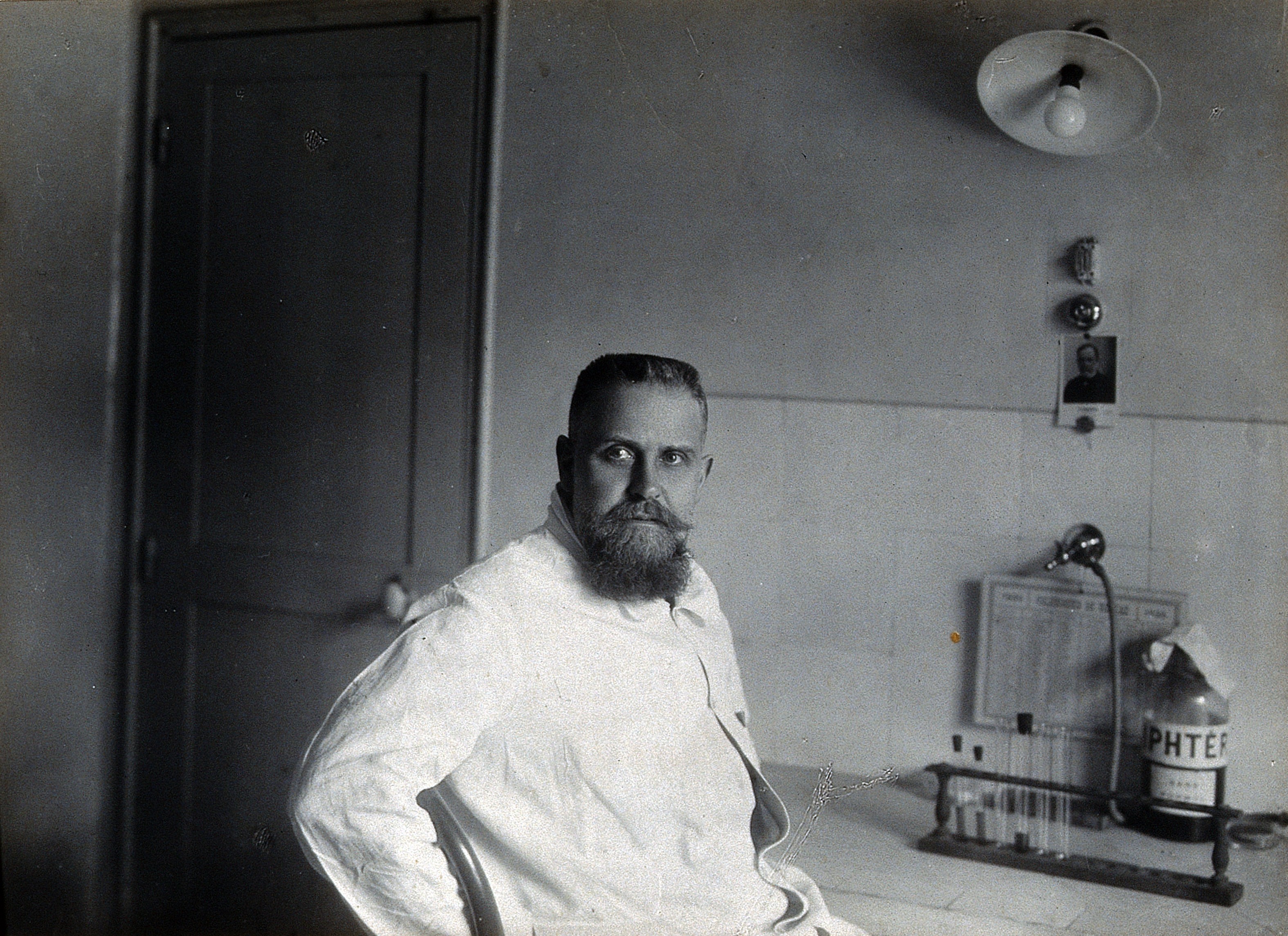
This defense usually starts with an immediate “innate” immune phase, where immune cells respond to general signs of distress. For instance, bacterial toxins damage cells and cause them to leak their contents, including DNA. When innate immune cells detect extracellular DNA, they initiate a response to this threat, recruiting more immune cells and inducing inflammation. If the innate response fails to destroy the intruder, the “adaptive” immune phase begins four to seven days later. B cells make antibodies that bind to specific targets on the pathogen, called antigens, which usually clear the toxins or infection with better precision.
After drawing blood from horses, Ramon isolated the antibodies by mixing them with the tetanus or diphtheria toxins to which they bind. The antibody-toxin complexes aggregated and formed a precipitate. The size of the precipitate corresponded to how much antibody the horses had produced.
In 1913, Ramon noticed that some horses had infected abscesses at the injection sites showing signs of inflammation: heat (calor), pain (dolor), redness (rubor), and swelling (tumor). Curiously, these horses made larger precipitates, suggesting higher antibody yields.
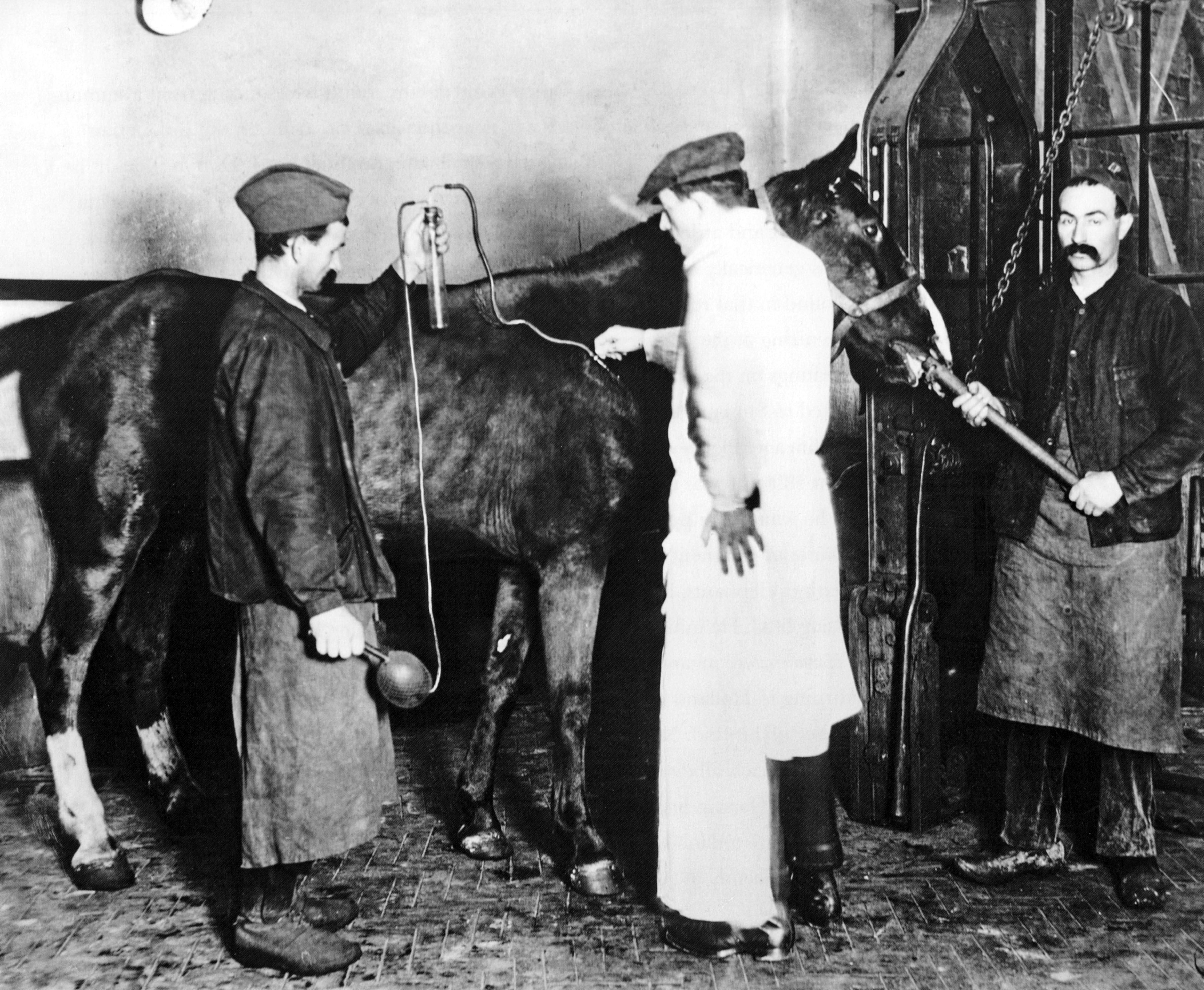
With our modern understanding of immunology — built upon discoveries that came after Ramon’s time — we can infer what caused the antibody boost. In horses that didn’t have an infection at the injection site, the innate immune system may have neutralized the toxins, reducing the need for B cells to proliferate and produce copious amounts of antibody. By contrast, in horses with infected abscesses, the immune system was fighting pathogens at the same time as the toxins, and a phenomenon called “heterologous immunity” could explain the elevation of antibody levels that ensued. Some immunologists argue that if two infections take place at once, the first infection triggers the immune system and gives it a “head start” on the second one. By the same logic, an infection in the horse’s abscess could have triggered the immune system, allowing it to detect the toxins more quickly and produce more antibodies.
But with immunology still in its infancy, Ramon was unaware of the immune dynamics taking place. He neglected, therefore, to explore the link between wounded horses and an enhanced immune response — at least for the moment.
A more pressing issue loomed. The First World War had broken out, and with Paris under siege, Ramon moved his serotherapy department to Toulouse to contribute to the defense effort. In order to produce enough antiserum against diphtheria and tetanus toxins to protect wounded soldiers, Ramon had to scale up operations by inoculating greater herds. Working tirelessly with 1,462 horses, his team churned out over 150,000 doses of antisera every month.
After the Armistice, Ramon returned to his research and shifted his focus from serotherapy to vaccines. This also required a shift toward animal research, for his bacterial toxins could not be administered to humans in their current form. For one thing, Ramon kept the toxin cultures heated to 38°C to ensure they remained stable at body temperature, but the warmth promoted the growth of bacterial contaminants that could cause harmful infections. He had to find a way to sterilize his cultures before administering them to people.
Ramon recalled learning in veterinary school that a small thimble of formaldehyde could sterilize a large quantity of milk, preventing it from turning without ruining its flavor. Applying this fixative to his toxin cultures, he found that it prevented contaminations without inhibiting antibodies from recognizing them.
One other problem remained: Bacterial toxins are dangerous. The diphtheria toxin kills healthy lung tissue and triggers fever and neck swelling, while the tetanus toxin stiffens muscle, causing lockjaw. To address this, Ramon heated his cultures to 55°C, warm enough to reduce their toxicity but not alter antibody binding. By 1923, he had perfected what he called his “anatoxines,” the molecules which later formed the basis for the first vaccines against diphtheria and tetanus.
But Ramon’s vaccines were far from ready. They didn’t provoke strong immune responses to the anatoxines, and his animal subjects produced too few antibodies. This prompted him to return to the curious finding that he had abandoned twelve years prior. Did horses with an abscess at the site of toxin injection make more antibodies because of the infection or the inflammation?
In 1925, Ramon determined that inflammation was key, and an infection, such as the ones in the horses’ wounds, wasn’t necessary to cause it. He created sterile abscesses by making superficial cuts in a horse’s skin and applying various chemicals, such as calcium chloride, cholesterol, or glycerin. Some substances created inflammation and heightened antibody levels more than others. Ramon dubbed these immune-helping stimulants “adjuvants,” from the Latin word adjuvare, meaning “to help.”
Ramon — encouraged by the results of his mixology — raided his pantry for yet other ingredients to test, including breadcrumbs, milk, olive oil, potato starch, and tapioca. Tapioca won out as the starchy substance bound strongly to bacterial toxins, slowly releasing them at the injection site and prolonging the immune response.
The Alum Age
Tapioca never featured in any human vaccines, though, because just one year after Ramon’s discovery, other researchers serendipitously discovered a superior adjuvant.
Immunologist Alexander Glenny and his team at the Wellcome Physiological Research Laboratories in London were using an array of chemicals to purify and concentrate diphtheria anatoxines.4 They employed a salt called potassium aluminium sulfate (alum), which bound to the anatoxines and caused them to precipitate out of the solution, forming an insoluble solid that Glenny’s team could inject into guinea pigs. Although Glenny’s team originally used alum only as a purifying agent, they soon realized it had adjuvant properties as well; vaccine formulations prepared with alum provoked stronger immune responses than ones purified with other chemicals.
Hours after vaccination, alum nodules formed at the injection site, and Glenny wondered if the alum — like Ramon’s tapioca — retained the anatoxines, releasing them slowly from the “depot” and lengthening the immune response. His team transplanted the nodules to other guinea pigs, which also produced antibodies against the anatoxines, confirming that the anatoxines clung to the alum precipitate for hours.
Vaccines containing alum were first administered to humans in 1932, and it has remained the favored adjuvant since then. By 1982, one-quarter of the world had received the diphtheria-tetanus-pertussis (DTP3) vaccine complemented with alum. Today, 51 percent of approved vaccine formulations contain alum, including vaccines for hepatitis A, hepatitis B, measles, mumps, rubella, and rotavirus.
Later research in the 1990s continued to explore alum’s efficacy, revealing that, in addition to serving as an antigen depot, the chemical may also provoke immunity by damaging tissue, causing cells to release their inner matter, such as uric acid. Since this chemical doesn’t appear outside cells except during tissue injury or gout, the immune system treats it as a danger signal, causing immune cells to rush toward the affected site.5 By instigating an antibody response, alum triggers the immune system to intercept extracellular virus particles or bacteria jumping between cells.
Subsequent work has explored how alum triggers signaling pathways, like the intracellular inflammasome, to release proinflammatory proteins known as cytokines. Research has also revealed how alum tweaks the immune response, favoring the production of antibodies that neutralize antigens.
However, since viruses can only replicate inside cells, some remain sheltered from antibodies. This may explain why alum-containing vaccines against herpes simplex virus (HSV) or human immunodeficiency virus (HIV) have failed. To address this deficiency, researchers developed additional adjuvants that predominantly activate killer immune cells, which destroy virus-infected cells. For instance, monophosphoryl lipid A, an FDA-approved adjuvant used to protect against shingles (varicella zoster virus), uses this approach.
Researchers continue to scout for new adjuvants capable of triggering new types of immune responses. The Precision Vaccine Program at the Boston Children’s Hospital screens immune cells for molecules that switch on inflammatory pathways, for example, and their latest candidate — called PVP-037 — broadly triggers antibody responses as well as killer immune cells.
Broadening our adjuvant repertoire to include varieties that tailor the immune response in different ways will be pivotal to improving vaccines, but alum and the five other FDA-approved adjuvants set a high bar for adjuvant standards. New candidates must measure up to or surpass current options in terms of efficacy, but they must not trigger too aggressive an immune response as that would make them unsafe.6 It’s an ongoing challenge to find adjuvants that hit the sweet spot in between.
Gaston Who?
Every year, over 100 million children across the globe receive a vaccination for diphtheria, tetanus, and pertussis. Just like James Phipps, many of them feel apprehensive about the ordeal. But unlike Phipps, they receive vaccines that reflect centuries of careful formulation and refinement, made possible by a meticulous scientist who spotted a connection between horse wounds and antibody titers.
Open any biology textbook to the page on vaccines and you are sure to find Edward Jenner’s portrait. He is universally lauded for setting vaccinology in motion and honored with everything from financial awards and honorary degrees to medals and commemorative statues. Had Jenner been alive when the Nobel Foundation formed, he would more than likely have been a shoo-in for the prize. Yet the researchers who discovered how to boost vaccine function with adjuvants have received comparatively little veneration.
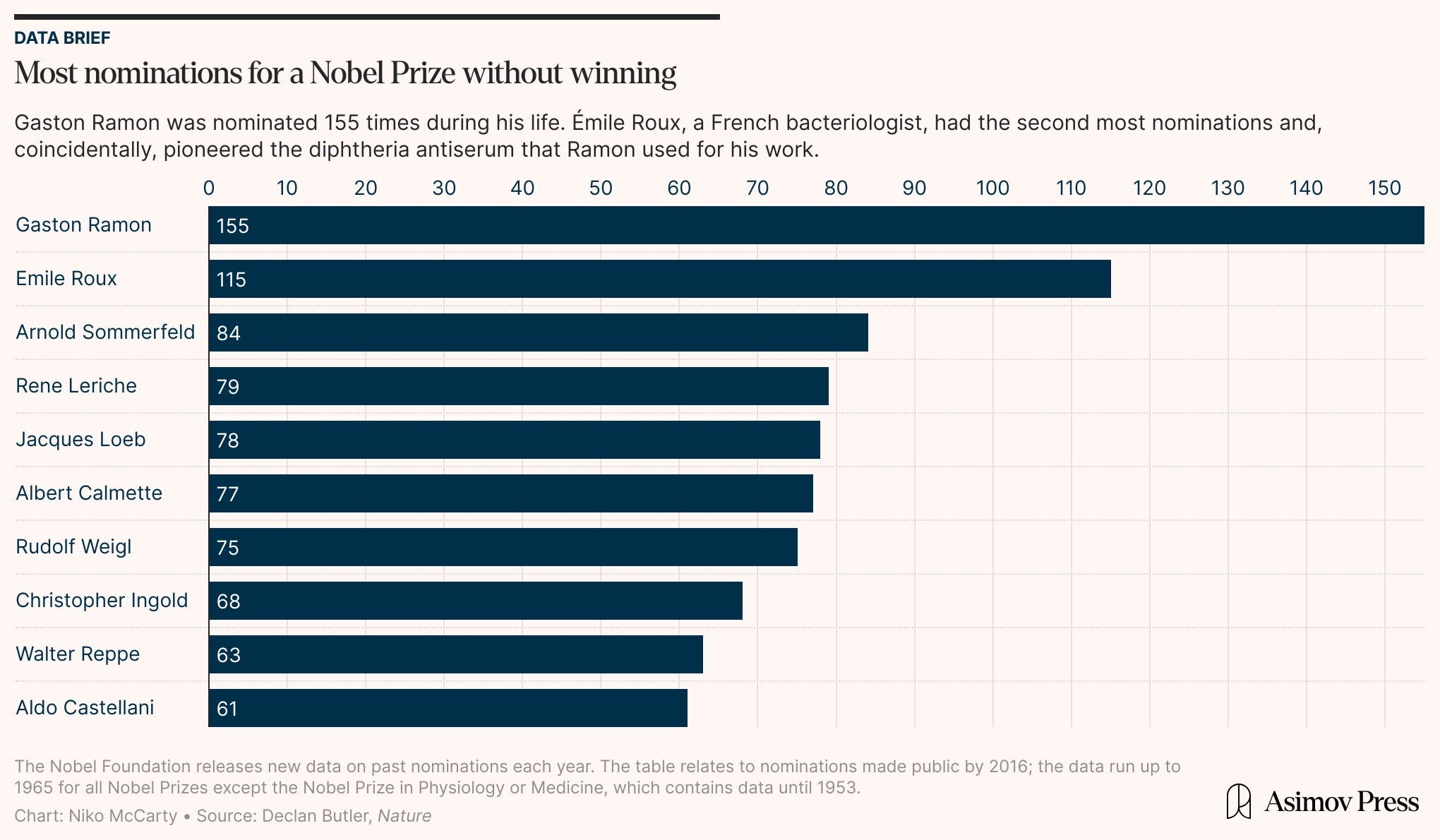
Gaston Ramon was nominated for a Nobel Prize 155 times during his lifetime — more than any other scientist — but never won, and few people alive today have heard of him. Alexander Glenny, too, felt overlooked for his contributions. That said, in Glenny’s biographical memoir, he is said to have conceded that “to praise anyone for doing a good job was really the worst of insults; it was the least that could be expected of him.”
A wounded horse, a pantry raid, and a serendipitous chemistry experiment led Ramon and Glenny to discover substances that provoke a stronger immune response to a vaccine. Their efforts have enhanced subunit vaccines and boosted the potency of nearly 30 other approved vaccine formulations. It’s high time to celebrate their fine-tuning of our defenses.
{{divider}}
Kamal Nahas is a researcher-turned-journalist based in Oxford, UK, who covers stories in biology, health, and technology.
Cite: Nahas, Kamal. “The Origins of Adjuvants.” Asimov Press (2025). DOI: https://doi.org/10.62211/83kj-77hg
Lead image by Ella Watkins-Dulaney.
Footnotes
- A later lithograph by Ernest Board paints a calmer picture — one in which a woman gently holds out James’ arm for Jenner to prick.
- Variolated individuals could also naturally transmit the virus to others through air droplets, which as previously mentioned may have contained a greater number of infectious virus particles than desiccated scab tissue.
- Mpox (formerly known as monkeypox) is another closely-related poxvirus that the smallpox vaccine protects against, and healthcare workers have used this shot on susceptible individuals during recent outbreaks that started in 2022.
- Independently of Ramon, Glenny prepared harmless derivatives of diphtheria and tetanus toxins fit for vaccination, but he called them “toxoids,” a term suggested by the German biochemist, Paul Ehrlich. To avoid switching between the two terms, I refer to them only as “anatoxines.”
- Recent research calls into question how important the slow release of antigen is to alum’s adjuvanticity, suggesting that the danger hypothesis may play a larger role.
- Some historic adjuvant candidates overstimulated the immune system, preempting their use in human vaccines. Complete Freund’s Adjuvant was developed in the 1930s and contained heat-killed Mycobacterium tuberculosis. The “cooked” bacterial components were potent stimulants in rabbits and guinea pigs but triggered allergic inflammation. Another example was a hydrocarbon-based adjuvant called pristane, which set off lupus-like symptoms in otherwise healthy mice.
Always free. No ads. Richly storied.
Always free. No ads. Richly storied.
Always free. No ads. Richly storied.